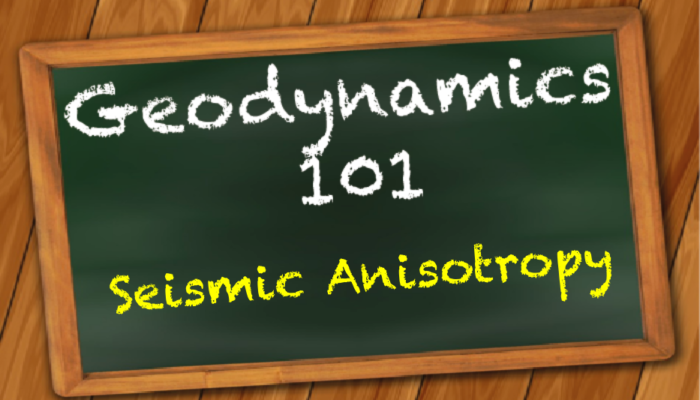
While we have sent several rovers to Mars, drilling down even just to the mantle of our own planet is a challenge that we are yet to overcome. How is it then that we know all these things about our planet’s interior? It turns out, we do not need to be inside the Earth to know what is happening there. Observations from geophysics and geochemistry can inform us about the processes and the properties of the Earth’s interior. Most of what we know about the deep Earth comes from our study of seismic waves that travel inside the Earth and are observed at the surface. A common method of studying the deformation and dynamics inside the earth involves the observation and mapping of seismic anisotropy (Savage, 1999; Long and Silver, 2009).
What is seismic anisotropy and how is it produced?
A medium through which seismic waves propagate is anisotropic if the waves have different velocities in different directions. The fast and the slow anisotropic directions are generally related to the kinematics of deformation. The directional dependence of seismic wave velocities can result from:
- Lattice-preferred orientation (LPO): alignment of anisotropic minerals in a preferred orientation (Karato et al., 2008) (Figure 1a), or
- Shape-preferred orientation (SPO): contrasting elastic properties are present adjacent to each other in the medium, for example, due to the layering of different materials, or alignment of cracks or melt pockets (Crampin and Lovell, 1991) (Figure 1b).
Figure 1 Seismic anisotropy due to (a) lattice-preferred orientation (LPO), and (b) shape-preferred orientation (SPO) (Image after Moore at al. (2004) from Garnero: http://garnero.asu.edu/research_images)
Where do we observe anisotropy in the earth?
Anisotropy has been observed in the crust, upper mantle, lowermost mantle (D” layer), and the inner core of the Earth (Long and Becker, 2010) (Figure 2).
Figure 2 Where have we observed anisotropy in the earth? (Image from Garnero: http://garnero.asu.edu/research_images)
Anisotropy in the upper crust is mostly due to stress-aligned cracks (Crampin and Lovell, 1991) while anisotropy in the lower crust is due to preferred orientation of minerals like the sheet-silicate micas (Christensen, 1966; Mainprice and Nicolas, 1989). When stress is applied, cracks perpendicular to the direction of maximum compression are closed preferentially, so that seismic waves travel faster along that direction (Crampin and Chastin, 2003). Higher seismic wave velocities are observed in the crust along faults (Hess, 1964) and along trends of orogenic belts (Lin and Schmandt, 2014).
Upper mantle anisotropy is primarily due to lattice-preferred orientation (LPO) of olivine (Karato et al., 2008), a mineral which makes up more than 60% of the mantle. Olivine is inherently anisotropic, reaching up to 18% of shear anisotropy (Mainprice et al., 2000) and seismic velocities are fastest along its a-axis [100] (Karato et al., 2008). For mantle flow in the horizontal plane, the olivine fast axes are generally aligned parallel to the direction of flow except under conditions of high stress and water content when the fast axes align perpendicular to the flow (Karato et al., 2008; Lynner et al., 2017).
Measurement of fast anisotropic directions in the mantle allows interpretations about the past tectonics and the current mantle dynamics. In the simplest case, anisotropic fast directions in the mantle would parallel plate motion (Karato et al., 2008). In the asthenosphere, current mantle flow determines the anisotropic fast direction whereas in the lithosphere, there could be fossil anisotropy due to past tectonic events. Near the mid-oceanic ridges, fast directions normally align with the spreading direction (Nowacki et al., 2012). Away from the ridges under normal oceanic crust, the fast directions generally correspond to plate motion (Long and Silver, 2009). On the other hand, the continents are generally associated with older crust and lithosphere that have undergone multiple episodes of deformation. The presence of multiple possible causes of anisotropy in the continents makes the interpretation of tectonics and dynamics from anisotropic signals more difficult (Long and Silver, 2009).
Most of the lower mantle is isotropic except just above the core-mantle boundary, i.e., the D” layer (Long and Becker, 2010). D” anisotropy could be due to lattice-preferred orientation of the lower mantle mineral post-perovskite (Karato, 1998) or due to shape-preferred orientation of aligned melt bodies (Kendall and Silver, 1998). In the solid inner core, the preferred alignment of crystals of iron results in anisotropy such that velocities are faster parallel to the axis of rotation (Karato, 1999). Although characterizing seismic anisotropy at greater depths such as that in the lower mantle and the inner core is more difficult, the measurement of anisotropy at these depths provides some constraints on the dynamics at these regions by encouraging inferences about the potential causes of the anisotropy.
Advances in the studies of seismic anisotropy can, therefore, help to better understand the processes that are happening inside our planet because of the cause-and-effect relationship between deformation and/or dynamics with the anisotropic fabric.
References: Christensen, N. I. (1966). Shear wave velocities in metamorphic rocks at pressures to 10 kilobars. Journal of Geophysical Research (1896-1977), 71(14), 3549–3556. https://doi.org/10.1029/JZ071i014p03549 Crampin, S., and Chastin, S. (2003). A review of shear wave splitting in the crack-critical crust. Geophysical Journal International, 155(1), 221–240. https://doi.org/10.1046/j.1365-246X.2003.02037.x Crampin, S., and Lovell, J. H. (1991). A decade of shear-wave splitting in the Earth’s crust: What does it mean? what use can we make of it? and what should we do next? Geophysical Journal International, 107(3), 387–407. https://doi.org/10.1111/j.1365-246X.1991.tb01401.x Hess, H. H. (1964). Seismic Anisotropy of the Uppermost Mantle under Oceans. Nature, 203(4945), 629–631. https://doi.org/10.1038/203629a0 Karato, S. (1998). Some remarks on the origin of seismic anisotropy in the D” layer. Earth, Planets and Space, 50(11), 1019–1028. https://doi.org/10.1186/BF03352196 Karato, S. (1999). Seismic anisotropy of the Earth’s inner core resulting from flow induced by Maxwell stresses. Nature, 402(6764), 871–873. https://doi.org/10.1038/47235 Karato, S., Jung, H., Katayama, I., and Skemer, P. (2008). Geodynamic Significance of Seismic Anisotropy of the Upper Mantle: New Insights from Laboratory Studies. Annual Review of Earth and Planetary Sciences, 36(1), 59–95. https://doi.org/10.1146/annurev.earth.36.031207.124120 Kendall, J.-M., and Silver, P. G. (1998). Investigating Causes of D″ Anistropy. In The Core-Mantle Boundary Region (pp. 97–118). American Geophysical Union (AGU). https://doi.org/10.1029/GD028p0097 Lin, F., and Schmandt, B. (2014). Upper crustal azimuthal anisotropy across the contiguous U.S. determined by Rayleigh wave ellipticity. Geophysical Research Letters, 41(23), 8301–8307. https://doi.org/10.1002/2014GL062362 Long, M. D., and Becker, T. W. (2010). Mantle dynamics and seismic anisotropy. Earth and Planetary Science Letters, 297(3–4), 341–354. https://doi.org/10.1016/j.epsl.2010.06.036 Long, M. D., and Silver, P. G. (2009). Shear Wave Splitting and Mantle Anisotropy: Measurements, Interpretations, and New Directions. Surveys in Geophysics, 30(4–5), 407–461. https://doi.org/10.1007/s10712-009-9075-1 Lynner, C., Long, M. D., Thissen, C. J., Paczkowski, K., and Montési, L. G. J. (2017). Evaluating geodynamic models for sub-slab anisotropy: Effects of olivine fabric type. Geosphere, 13(2), 247–259. https://doi.org/10.1130/GES01395.1 Mainprice, D., Barruol, G., and Ismaïl, W. B. (2000). The Seismic anisotropy of the Earth’s mantle: From single crystal to polycrystal. In S. Karato, A. Forte, R. Liebermann, G. Masters, and L. Stixrude (Eds.), Geophysical Monograph Series (Vol. 117, pp. 237–264). American Geophysical Union. https://doi.org/10.1029/GM117p0237 Mainprice, D., and Nicolas, A. (1989). Development of shape and lattice preferred orientations: Application to the seismic anisotropy of the lower crust. Journal of Structural Geology, 11(1–2), 175–189. https://doi.org/10.1016/0191-8141(89)90042-4 Nowacki, A., Kendall, J.-M., and Wookey, J. (2012). Mantle anisotropy beneath the Earth’s mid-ocean ridges. Earth and Planetary Science Letters, 317–318, 56–67. https://doi.org/10.1016/j.epsl.2011.11.044 Savage, M. K. (1999). Seismic anisotropy and mantle deformation: What have we learned from shear wave splitting? Reviews of Geophysics, 37(1), 65–106. https://doi.org/10.1029/98RG02075 *Garnero: http://garnero.asu.edu/research_images/