“Girls into Geoscience” is a UK-based outreach initiative aiming at encouraging female (and female identifying and non-binary) to pursue a career in Geosciences. I had the pleasure to interview the founders of this initiative, Prof. Sarah Boulton and Dr. Jodie Fisher, and discuss about their experiences, how the landscape of student recruitment has changed over the years and the challe ...[Read More]
Into the world of Girls into Geoscience: inspiring the future generation of female geoscientists
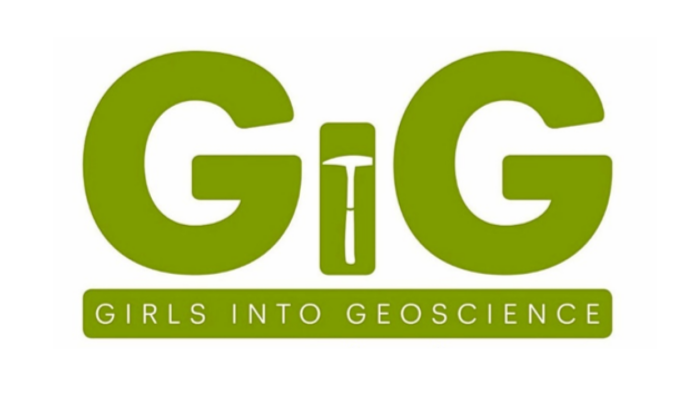