In November last year (see this post), we promised to provide you an update of what happened with Antarctic sea ice during the year 2023 – a year of an exceptionally low extent. In this post, we try our best to discuss the conditions that lead to (part of) the recent loss in Antarctic sea ice, including atmospheric and ocean processes. How sea ice usually works… One of the ways scientists h ...[Read More]
You thought it was over? Here’s more on the 2023 Antarctic sea-ice extent record low
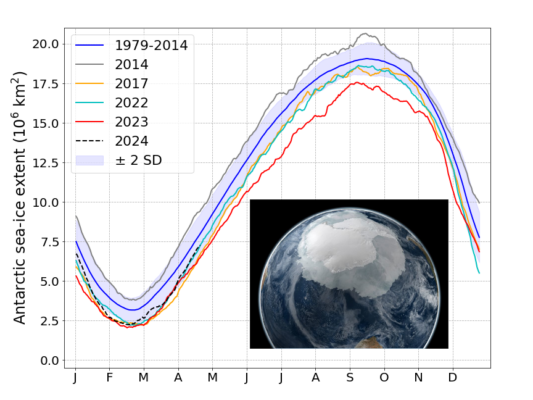