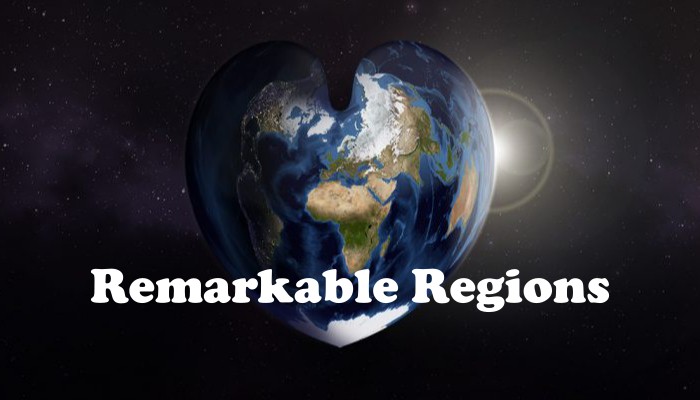
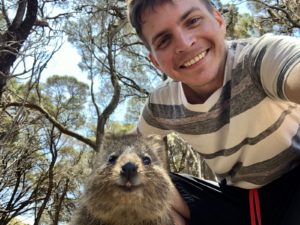
Derek Neuharth, PhD student in the Geodynamic Modelling section of GFZ Potsdam. And a quokka.
2020 left your head spinning? This week Derek Neuharth from GFZ Potsdam introduces us to the spinning microplates that can be found in extensional plate boundaries such as the East African Rift System and the East Pacific Rise.
Divergent plate boundaries
Microplates are enigmatic features that form in the boundaries between tectonic plates. Generally, plate boundary dynamics are divided into three types: 1) convergent boundaries where plates collide (e.g., Andes subduction zone; Sobolev & Babeyko, 2005), 2) transform boundaries where plates grind past each other (e.g., San Andreas fault; Powell & Weldon, 1992), or 3) divergent boundaries where plates move apart (e.g., mid-ocean ridges). However, plate boundaries are not static and they evolve over time. In the case of divergent boundaries, forces can pull apart continental crust and form rifts. As spreading continues, opposing rifts can meet and split apart the continents, eventually leading to oceanic spreading and the formation of entirely new oceans and plate boundaries. Of course, the crust is not homogenous and rifts responding to the same far-field forces therefore do not form in perfectly straight lines. Also, rifts can initiate hundreds of kilometres apart both along and perpendicular to strike (e.g., formation of the South Atlantic; Heine et al., 2013).
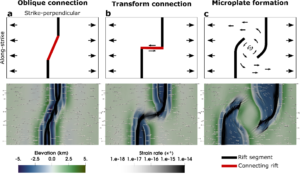
Figure 1: Conceptual (top) and numerical (bottom) models showcasing three types of rift linkage. a) Connection of rifts through an oblique fault at small strike-perpendicular offsets. b) Transform connection of rifts offset at an intermediate offset. c) Formation of a continental microplate at large (300 km) initial rift offsets.
As offset rifts responding to the same forces propagate forward, they are likely to interact and link. This linkage can happen in a few ways (Fig. 1), and one important factor controlling how they link is their strike-perpendicular offset (Allken et al., 2011, 2012; Gerya, 2013; Neuharth et al., in review). If the two segments are relatively close together when they meet, an oblique fault segment connects them (Fig. 1a). At higher offsets, the rifts propagate farther along-strike before interacting. In this case, the connecting fault is more oblique and rift segments connect through a transform fault (Fig. 1b). When the offset is even further increased, the rift segments are too far apart to properly connect and instead they overlap. As extension continues, the rigid block between the overlapping segments, known as a microplate, is forced to rotate to accommodate lateral extension of the crust (Fig. 1c). It is in this relatively short (geologically speaking) time where rifts meet, interact, and compete that active microplates exist.
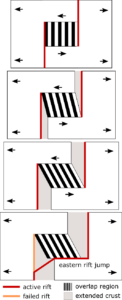
Figure 2: Simplified conceptual model of microplate rotation assuming purely orthogonal extension and no rift propagation.
What causes microplate rotation?
Although there are a few proposed reasons for microplate rotation (e.g., Neves et al., 2003; Schouten et al, 1993), one explanation is that rotation is driven by drag forces along the edges of the microplate (Schouten et al., 1993; Katz et al., 2006). Along active rift segments, the crust’s extent is increased either through crustal/lithospheric thinning or the formation of oceanic crust. As microplates exist in the specific case where two rifts have overlapped instead of connected, the rigid block in the overlapping region is still attached to both sides of the tectonic plate. Because of this, crustal extension at the offset rift segments outside the overlapping region force the two sides to move in opposite directions (Fig. 2) These generally orthogonal, opposing, velocities on the top and bottom of the microplate rotate the rigid block. This rotation continues until, eventually, one rift becomes dominant and propagates forward, connecting to the opposing rift through a rift jump. At this time, the leftover weaker rift segment no longer accommodates extension and fails, leaving the now inactive microplate attached to the side opposite the rift jump direction.
Microplate examples
Active extensional microplates are seen today along divergent plate boundaries in oceanic (e.g., Easter Island microplate; Naar & Hey, 1991) and continental settings. In oceanic settings, microplates are short-lived events that occur along mid-ocean ridges as spreading centres overlap (Keary et al., 2009; Schouten et al., 1993). The Victoria microplate is an example of an active continental microplate (Fig. 3), where rift arms have been guided by weak suture zones to encompass a rigid, rotating, block (Glerum et al., 2020).
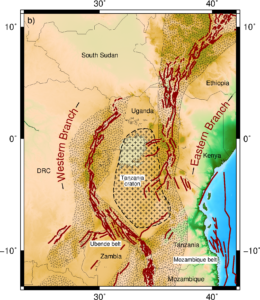
Figure 3: Map view of the Victoria microplate in the East African Rift System. Modified from Glerum et al. (2020), including ETOPO1 topography and active faults from the Global Earthquake Model Foundation database. Mobile belt outlines from Ring (2014), craton from Nyblade & Brazier (2002).
As active continental microplates exist today, one would expect evidence of remnant ones. When examining continental margins, the margins left from previous ocean-forming rifting events (e.g., coastlines of Africa and South America), seismic data shows regions of locally thick continental crust surrounded by oceanic or thinned continental crust. One example is the Flemish Cap (Fig. 4), located off the coast of Newfoundland, a region of thick crust (20-30 km) that formed during the break-up of the supercontinent Pangea. The Flemish Cap is surrounded by thinned continental crust from a failed rift in the Orphan basin to the west and an ocean-forming eastern rift (Welford et al., 2012, 2020). It rotated in a clockwise direction (Sibuet et al., 2007) until an eastern rift jump left it attached to North America.
In the CRYSTALS group we have set up numerical models to investigate the development of continental microplates from initiation until continental break-up (Neuharth et al., in review). Given an initial rift orientation representative of the Iberian-North American rifting event, we found that modelled microplates are remarkably similar to the Flemish Cap (Fig. 4). Mechanically, the modelled microplate is rotated clockwise until an eastern rift jump leaves it attached to the western margin. The microplate is surrounded by oceanic crust to the west from a failed rift, analogous to the one found in the Orphan Basin, and an ocean-forming rift to the east. Additionally, the size and geometry of the modelled microplate closely resemble the Flemish Cap.
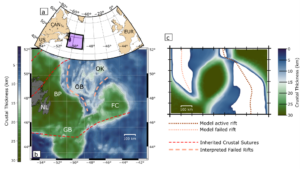
Figure 4: a) Location of the Flemish Cap. b) Crustal thickness map of the Newfoundland margin (using methodology of Welford et al., 2012), with abbreviations BP, Bonavista Platform; FC, Flemish Cap; GB, Grand Banks; NL, Newfoundland; OB, Orphan Basin; OK, Orphan Knoll. c) Crustal thickness map of the modelled microplate. Modified from Neuharth et al. (in review).
Future work
For microplate development, it is important to not only consider what occurs inside the Earth, but also the processes that change the surface. Recently, many tectonic codes have been coupled with surface processes codes (e.g., Underworld with Badlands, Beucher et al., 2018; Sopale with FastScape, Beucher & Huismans, 2020) to test the interactions between margin evolution and erosion/deposition. Within the CRYSTALS group, I have been working on coupling the surface processes code FastScape (Braun & Willett, 2013; Yuan et al., 2019a; Yuan et al., 2019b) with the tectonic code ASPECT (Heister et al., 2017; Kronbichler et al., 2012) and applied this to a microplate model as exemplified by this movie:
While this setup does not include stratigraphy, future modelling will track where sediment is deposited to examine basins that form around developing microplates and apply this to real-world remnant microplates.
Although many studies have examined plate boundary dynamics and rift linkage, there is so much complexity left to investigate. Whether it be how directional changes in extension may affect rift linkage and microplate development (Zwaan et al., 2016), or examining the effect of melting processes on suspected microplates in magmatically active regions (e.g. Sao Paulo Plateau; Scotchman et al., 2010), or how varying erosion rates may influence rift dynamics; there remain many processes left to explore, and I am looking forward to the development and discoveries made by the scientific community in the future.
References Allken, V. et al. (2011). Journal of Geophysical Research: Solid Earth, 116(10), 1–15. Allken, V. et al. (2012). Geochemistry, Geophysics, Geosystems, 13(5), 1–18. Beucher, R., & Huismans, R. S. (2020). Geochemistry, Geophysics, Geosystems, 21(5). Beucher, R. et al. (2018). ASEG Extended Abstracts, 1. Braun, J., & Willett, S. D. (2013). Geomorphology, 180–181, 170–179. Gerya, T. (2013). Physics of the Earth and Planetary Interiors, 214, 35–52. Glerum, A. et al. (2020). Nature Communications, 11(1), 1–15. Heine, C. et al. (2013). Solid Earth, 4(2), 215–253. Heister, T. et al. (2017). Geophysical Journal International, 210, 833–851. Keary, P., Klepeis, K. A., & Vine, F. J. (2009). Global Tectonics. John Wiley & Sons, LTD. Kronbichler, M. et al. (2012). Geophysical Journal International, 191. Naar, D. F., & Hey, R. N. (1991). Journal of Geophysical Research, 96(B5), 7961–7993. Neuharth, D., Brune, S., Glerum, A., Heine, C., & Welford, J. K. (in review). Formation of continental microplates through rift linkage: Numerical modelling and its application to the Flemish Cap and Sao Paulo Plateau. Geochemistry, Geophysics, Geosystems. Neves, M. C. et al. (2003). Journal of Geophysical Research: Solid Earth, 108(B4). Powell, R. E., & Weldon, R. J. (1992). Annual Review of Earth and Planetary Sciences. Vol. 20, 431–468. Schouten, H. et al. (1993). Journal of Geophysical Research, 98, 6689–6701. Scotchman, I. C. et al. (2010). Petroleum Geology Conference Series, 7, 855–866. Sibuet, J. C., et al. (2007). Geological Society Special Publication, 282, 63–76. Sobolev, S. V, & Babeyko, A. Y. (2005). Geology, 33(8), 617–620. Welford, J. K. et al. (2020). Geophysical Journal International, 221(1), 37–59. Welford, J. K. et al. (2012). Journal of the Geological Society, 169(4), 405–420. Yuan, X. P. et al. (2019). Journal of Geophysical Research: Earth Surface, 124(6), 1346–1365. Yuan, X. P. et al. (2019). Earth and Planetary Science Letters, 524, 115728. Zwaan, F. et al. (2016). Tectonophysics, 693, 239–260.