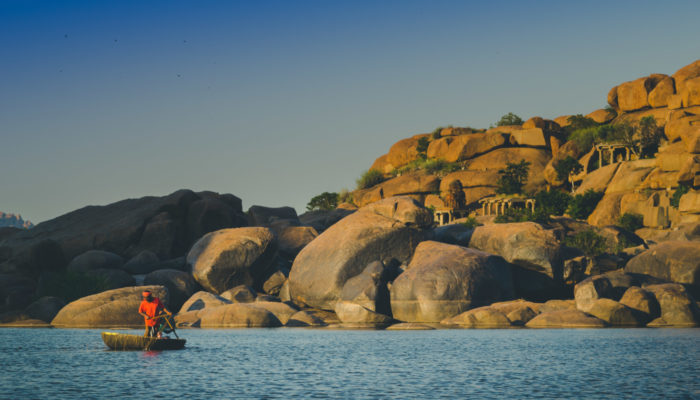
One hundred years back, Leopold Kober first introduced the term “Kratogen”. With time, the concept of kratogen has evolved, and they are now known as cratons. In this week’s news and views, Jyotirmoy (@GeophyJo), a PhD student from the Indian Institute of Science revisits the history of craton science: how the craton concept has evolved and what are the modern problems related to them.
…cratons have a life and tectonic personality of their own. Ali Mehmet Celâl Şengör
Etymology of craton
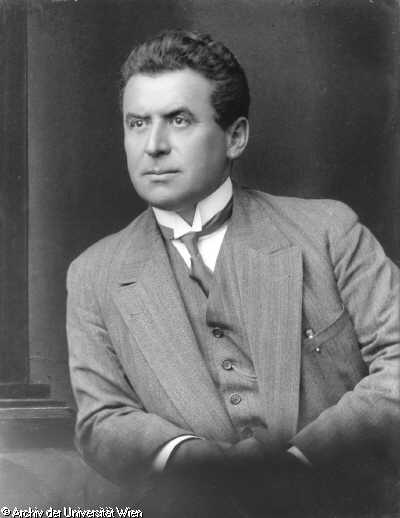
Fig. 1: Leoplod Kober, source: https://gedenkbuch.univie.ac.at
J.D Dana was one of the first geologists to provide a geomorphological basis of a continent consisting of mountain ranges at the continental border facing oceans and a stable continental interior (Dana, 1863). This hypothesis was purely based on geological observation of American continents. However, large mountain ranges in Asia’s interior was a certain misfit to this stable continental interior proposition. In 1900, Emile Hung came up with a new hypothesis of geosyncline and stable continental parts (Hung, 1900) that could better fit the ’Asiatic structure’ as this new concept was not strictly a geographical position within a continent. Austrian geologist Leopold Kober was the first person to conceptualize earth’s crust into two geomorphic divisions, namely orogen and kratogen (Kober, 1921).
The word kratogen originated from the combination of Greek words κρατos (strength) and γνσis (way of birth). So, the term was introduced by Kober to indicate parts of the continent that were born strong. In 1936, Hans Stille reintroduced stable continents as kraton instead of kratogen (Stille, 1936). In 1944, Marshall Kay introduced ‘craton’ in English vocabulary, which became more popular than kratogen (Kay, 1947). Kober, the pioneer of the kratogen concept, had argued, “We accept this designation here, though linguistically it does not fit into the nomenclature given here, because with the suffix -on we designate a time unit, whereas with -gen, a space unit. A more correct form would have been kraten” (Şengör, 1999).
Definition of a craton
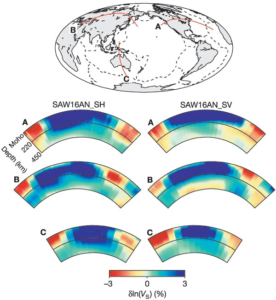
Fig. 2: Cratonic lithosphere are shown in dark blue regions. Thickness does not exceed beyond ~250 km. Figure from Gung et al., 2003.
The definition of craton has evolved gradually. In the mid-1900s, Stille defined cratons as parts of the earth’s crust that does not undergo penetrative deformation (alpinotype) but non-penetrative deformation (germanotype), which creates blocky structures with moderate strain and metamorphism (Stille, 1940). Kay added these areas to be primarily silicic (Kay, 1947). Stille added another terminology as quasicratons, which indicates addition of more silicic materials to an existing craton during a later orogeny(Stille, 1940).
A more modern definition of cratons appeared during the mid-1970s when Jordan proposed his tectosphere hypothesis (Jordan, 1975, 1978). He defined the cratons as the continental tectosphere that are at least 400 km thick and may go to 700 km occupying the whole upper mantle (Jordan, 1978). These regions have very low heat flux, and the mode of heat transfer is only conduction. Gung et al. (2003) contradicted the proposition of a 400 km thick tectosphere. Using radial anisotropy data, they showed that Jordan’s tectosphere should not be more than 250 km thick (Fig. 2). Scott King defined the Archaean cratons as “relatively flat, stable regions of the crust that have remained undeformed since the Precambrian, forming the ancient cores of the continents” King (2005). The subcontinental lithospheric mantle of these cratons is termed as roots. However, Jordan argued using the term ‘root‘ as a misnomer because root will indicate continents are grounded; rather, he suggested ‘keel‘ as more appropriate in this context (Yoshida & Yoshizawa, 2020).
Cratons’ long term survival:
Over the 100 years, more and more complexities were added to the craton related problems. One of the most debated topics in this context is the long term survival of cratons (Artemieva & Mooney, 2002; Beall et al., 2018; Cooper et al., 2006; Foley, 2008; Gerya, 2014; Jordan, 1975, 1978; Lenardic & Moresi, 1999; King, 2005; Lee et al., 2005, 2011; Lenardic et al., 2000, 2003; O’Neill et al., 2008; Paul et al., 2019, Paul and Ghosh 2020, Perchuk et al., 2020; Sleep, 2003; Wang et al., 2014, 2018; Yoshida, 2010, 2012; Yoshida & Yoshizawa, 2020). Because of tectonic recycling, any non-cratonic lithosphere is destroyed within a few hundred million years, whereas the cratonic lithosphere has often remained stable for more than 3 billion years. Understanding this tectonic inertness of cratons has been considered as one of the grand challenges of geodynamics, “How the continents, including their deep roots, nearly rigid interiors, and deforming margins accommodate tectonic plate motions is a future grand challenge for geodynamics” (Olson et al., 2010).
A hypothesis of neutrally buoyant and highly viscous craton seems to have been well established to explain their stability. In the last 40 years, a number of geodynamic models have been developed in 2-D box set up , 3-D box set up, 3-D spherical set up in restricted domain, and global 3-D spherical set up (Lenardic & Moresi, 1999; Lenardic et al., 2000, 2003; O’Neill et al., 2008; Paul et al., 2019, Paul and Ghosh 2020, Sleep, 2003; Wang et al., 2014, 2018; Yoshida, 2010, 2012) to estimate the viscosity of cratons that can support their long term stability. Most studies suggested that the viscosity of craton needs to be at least 100-1000 times more than their surroundings to support the long term survival of cratons (Fig. 3).
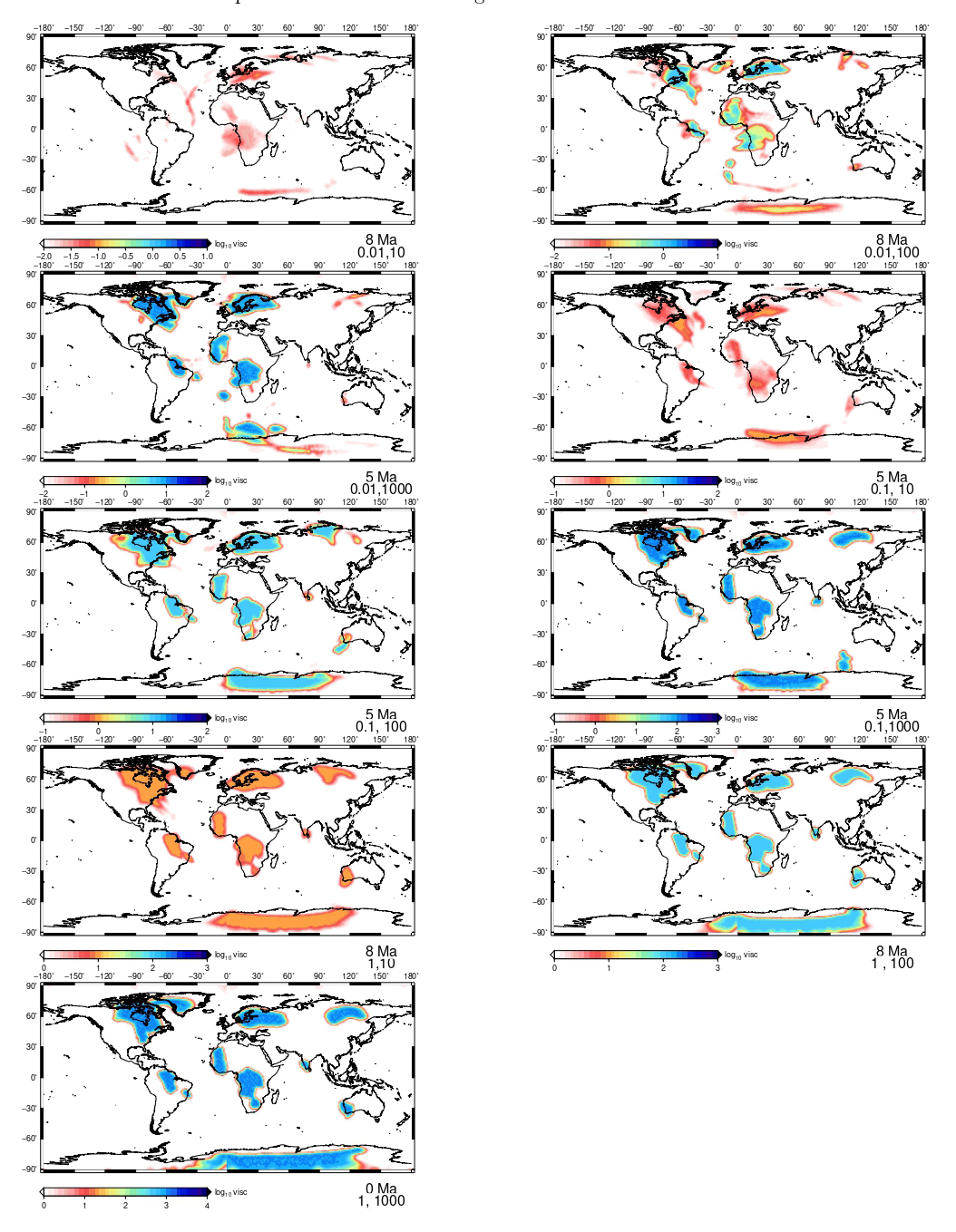
Fig. 3: Evolution of cratons has been modeled for last ~ 400 Ma using different craton and asthenosphere viscosity. When cratons are at least 100 times more viscous than the surroundings, and the asthenosphere viscosity is more or equal to 10^20 Pa-s (0.1), cratons remain stable for more than 400 Ma. Figure from Paul and Ghosh, 2020.
However, using a non-Newtonian viscosity, Wang et al. (2014) showed that only 10 times viscosity contrast between the craton and surroundings is enough to achieve tectonic stability (Fig. 4).
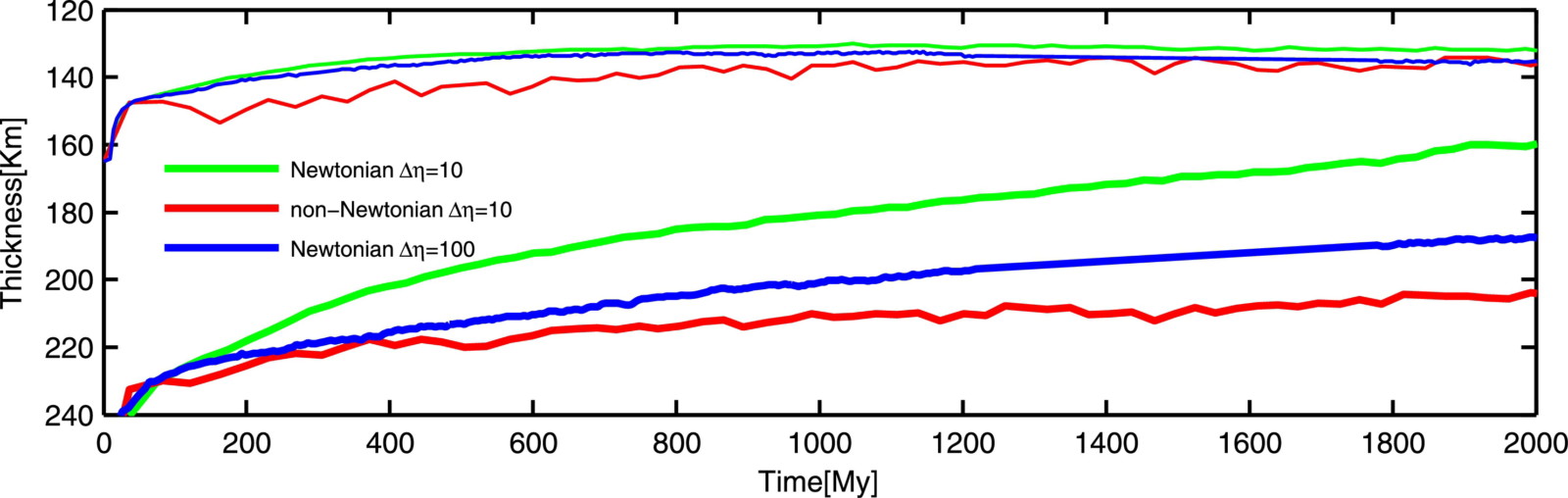
Fig. 4: With low non-Newtonian viscosity contrast craton root remain thick over 2000 My (From Wang et al., 2014)
Lenardic et al. (2000) proposed that the presence of a weak crumpled zone surrounding cratons can also contribute to the survival of cratons. Yoshida (2012) supported this argument using 3-D spherical convection models (Fig. 5). Artemieva and Mooney (2002) advocated that the craton thickness also helps in their survival, and this was tested numerically by Paul et al. (2019). Paul and Ghosh (2020) also argued that the cratons’ size could be crucial for their survival.
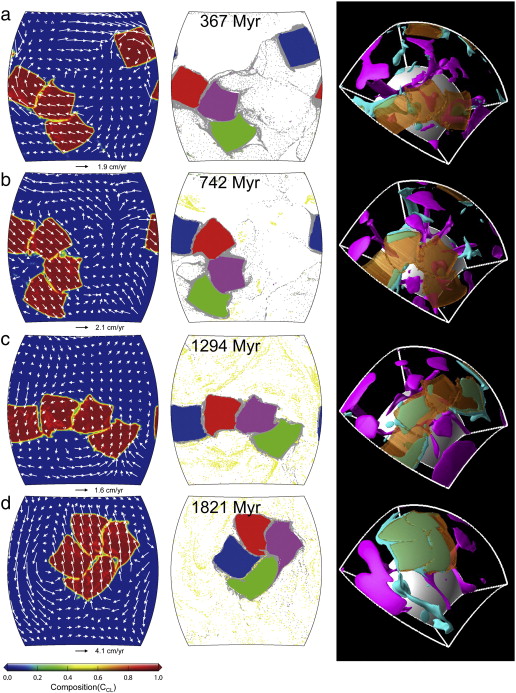
Fig. 5: Viscosity of weak layer surrounding the continental lithosphere is 10^6 times lesser. Due to such weak layer surrounding continental blocks, they remained stable for ~2 by. Figure from Yoshida 2012.
Destruction of cratons:
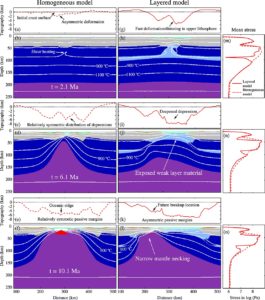
Fig 6. The weak MLD layer deforms the lithosphere above it (right panel), but slows down deformation of the lithosphere beneath it Figure from Liao et al. 2013.
Even after remaining tectonically quiet for more than a few billion years, some cratons are slowly crumbling now. For example, the eastern part of the North China craton is reported to have been destroyed in the Triassic (Xu 2001, Zhu et al., 2012). Several ad-hoc mechanisms have been proposed as potential reasons for craton destruction, such as metasomatism, magmatic infiltration, subduction-related processes, and delamination (Lee et al., 2011). The discovery of mid-lithospheric discontinuity (MLD) in the last decade has opened a new avenue to understand the craton destruction process (Abt et al., 2010; Aulbach et al., 2017, Liao et al., 2013; Liao & Gerya, 2014; Wang et al., 2017, Yuan & Romanowicz, 2010). The study by Liao et al., (2013) is one of the first attempts to numerically model the effect of weak MLD on the craton dynamics (Fig. 6). In fact, many studies now relate the destruction of the North China craton to a weak MLD (Liu et al., 2018). Dislocation of African cratonic root has also been connected to the presence of MLD underneath the African craton (Wang et al., 2017).
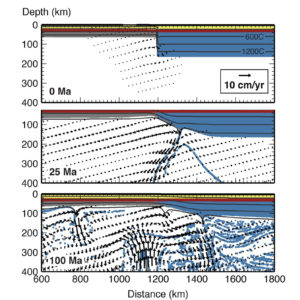
Fig. 7: Edge driven convection destroying thick cratonic lithosphere. Figure from Currie and van Wijk, 2016
Edge-driven convection along the thick cratonic edge is also thought to be one of the primary reasons for craton destruction (Currie and van Wijk, 2016). In the Wyoming craton, a flat slab induced edge-driven convection has been proposed to be its cause of destruction (Dave and Ali, 2016).
Cratons often interact with the mantle plume, which is another potential factor for destroying cratonic roots. Kumar et al. (2007) proposed that the eruption of the Reunion plume underneath the Indian lithosphere might have thinned down the Indian craton. Apart from just thermal delamination, plumes could also provide magmatic fluid, which can react with the cratonic roots to make them weaker. Such a long time reaction may incise the cratonic roots into smaller units resulting the demise of a craton (Fig. 8) (Foley 2008).
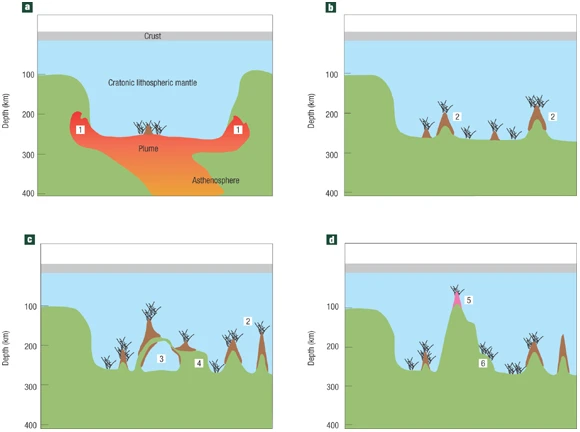
Fig 8. Destruction of craton due to plume eruption and by melt-fluid incision. Figure from Foley 2008.
Resolving the tectonic paradigm in the early earth:
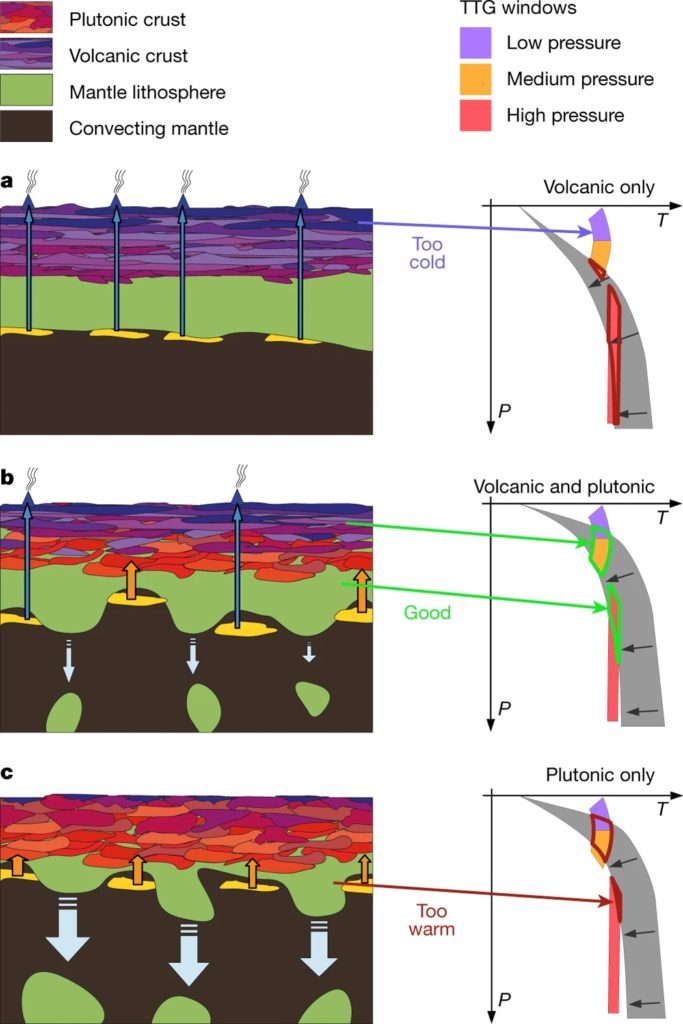
Fig. 9: Different modes of tectonism in Archaean earth and mechanism for TTG (equivalent composition of cratons) formation. Figure from Rozel et al. 2020.
At ~3 Ga, when cratons were forming, the tectonic regime of the earth is not clearly known. It was probably undergoing a change from the stagnant lid mode to the mobile lid mode of tectonics at around this time. A few studies have shown that cratonic composition can be formed from a squishy-lid tectonic regime in the early earth (Fig. 9) (Rozel et al., 2017).
However, to make cratons thicker than the average non-cratonic lithosphere, horizontal compression might be required. Beall et al., 2018 suggested this compression resulted from the massive stress originated during the lid breaking event at ~3 Ga when earth’s tectonism was shifting from the stagnant lid mode to the mobile lid mode (Fig. 10). This mechanism could also explain internal suture zones of cratons, which could become weak MLDs later. On the other hand, Wang et al., 2018 argued this thickening is rather gradual and consisted of two stages: tectonic shortening and gravitational thickening. Though the exact nature of the thickening of cratons has remained controversial, more research works will bring up new ideas and hypotheses in the near future.
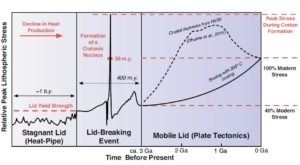
Fig. 10: Peak stress was reached at ~ 3 Ga and cratons got thickened during this time. Figure from Beall et al., 2018.
Cratons are the only preserved evidence of the earth’s early geodynamic history. To resolve the nature of the Precambrian geodynamics, understanding the formation and stabilisation mechanisms of craton remains as the most essential tool. Within 100 years, the geodynamics community has made reasonable progress in the craton and early earth related problems. This is going to set the ground for the next level of scientific exploration in the upcoming years.
References: Abt, D. L., Fischer, K. M., French, S. W., Ford, H. A., Yuan, H., & Romanowicz, B., 2010. North American lithospheric discontinuity structure imaged by Ps and Sp receiver functions, Journal of Geophysical Research: Solid Earth, 115, B09301, doi:10.1029/2009JB006914. Artemieva, I. & Mooney, W., 2002. On the relations between cratonic lithosphere thickness, plate motions, and basal drag, Tectonophysics, 358(1), 211-231. Aulbach, S., Massuyeau, M., & Gaillard, F., 2017. Origins of cratonic mantle discontinuities: A view from petrology, geochemistry and thermodynamic models, Lithos, 268, 364-382. Beall, A., Moresi, L., & Cooper, C., 2018. Formation of cratonic lithosphere during the initiation of plate tectonics, Geology, 46(6), 487-490. Cooper, C., Lenardic, A., Levander, A., & Moresi, L. N., 2006. Creation and preservation of cratoniclithosphere: seismic constraints and geodynamic models, in Archean Geodynamics and Environments, pp. 75-88, eds K Benn, J. M. & Condie, K. C., American Geophysical Union, Geophysical Monograph Series. Currie, C.A. and van Wijk, J., 2016. How craton margins are preserved: Insights from geodynamic models. Journal of Geodynamics, 100, pp.144-158. Dave, R. and Li, A., 2016. Destruction of the Wyoming craton: Seismic evidence and geodynamic processes. Geology, 44(11), pp.883-886. Dana, J. D., 1863. Manual of Geology: Treating of the principles of the science with special reference to American geological history, Theodore Bliss, Philadelphia Foley, S. F., 2008. Rejuvenation and erosion of the cratonic lithosphere,Nature geoscience,1(8),503. Gung, Y., Panning, M., & Romanowicz, B., 2003. Global anisotropy and the thickness of continents,Nature,422 (6933), 707–711. Hung, E., 1900. Gosynclinaux et les ares continentales. contribution ltude des transgressions et regressions marines, Bulletin de la Socit gologique de France, 28 (3), 617–711. Jordan, T., 1975. The continental tectosphere, Reviews of Geophysics, 13 (3), 1–12. Jordan, T., 1978. Composition and development of the continental tectosphere, Nature, 274 (5671), 544–548. Kay, M., 1947. Geosynclinal nomenclature and the craton, AAPG Bulletin,31 (7), 1289–1293. King, S., 2005. Archean cratons and mantle dynamics, Earth and Planetary Science Letters, 234 (1), 1–14. Kober, L., 1921.Der Bau der Erde, Gebr ̈uder Borntraeger. Kumar, P., Yuan, X., Kumar, M. R., Kind, R., Li, X., & Chadha, R., 2007. The rapid drift of the indian tectonic plate,Nature,449 (7164), 894–897 Lee, C.-T. A., Lu, P., & Chin, E. J., 2011. Building and destroying continental mantle, Annual Review of Earth and Planetary Sciences, 39, 59-90. Lenardic, A. & Moresi, L. N., 1999. Some thoughts on the stability of cratonic lithosphere: e ects of buoyancy and viscosity, Journal of Geophysical Research: Solid Earth, 104(B6), 12747-12758. Lenardic, A., Moresi, L. N., & Muhlhaus, H., 2000. The role of mobile belts for the longevity of deep cratonic lithosphere: the crumple zone model, Geophysical Research Letters, 27(8), 1235-1238. Lenardic, A., Moresi, L. N., & Muhlhaus, H., 2003. Longevity and stability of cratonic lithosphere: insights from numerical simulations of coupled mantle convection and continental tectonics, Journal of Geophysical Research: Solid Earth, 108(B6), https://doi.org/10.1029/2002JB001859. Liao, J. & Gerya, T., 2014. Infuence of lithospheric mantle stratication on craton extension: Insight from two-dimensional thermo-mechanical modeling, Tectonophysics, 631, 50-64. Liao, J., Gerya, T., & Wang, Q., 2013. Layered structure of the lithospheric mantle changes dynamics of craton extension, Geophysical Research Letters, 40(22), 5861-5866. Liu, L., Morgan, J. P., Xu, Y., & Menzies, M., 2018. Craton destruction 1: Cratonic keel delamination along a weak mid lithospheric discontinuity layer, Journal of Geophysical Research: Solid Earth,123(11), 10-040. Olson, P., Bercovici, D., Buffet, B., Carlson, R., Flesch, L., Morgan, J.P., Roy, M, & Valencia, D., 2010. Grand challenges in Geodynamics, Outstanding geodynamics problems and emerging research opportunities for the Earth Sciences, National Science Academy, Wahsington. O'Neill, C.J., Lenardic, A., Griffin, W.L. and O'Reilly, S.Y., 2008. Dynamics of cratons in an evolving mantle. Lithos, 102(1-2), pp.12-24. Şengör, A.M.C., 1999. Continental interiors and cratons: any elation?. Tectonophysics, 305(1-3), pp.1-42. Sleep, N. H., 2003. Survival of Archean cratonal lithosphere, Journal of Geophysical Research: Solid Earth, 108(B6), 2302, doi:10.1029/2001JB000169 Stille, H., 1936. Tektonische Beziehungen Zwischen Nord-Amerika und Europa, editeur inconnu. Wang, H., van Hunen, J., Pearson, D. G., & Allen, M. B., 2014. Craton stability and longevity: The roles of composition-dependent rheology and buoyancy, Earth and Planetary Science Letters, 391, 224-233. Wang, Z. & Kusky, T. M., 2019. The importance of a weak mid-lithospheric layer on the evolution of the cratonic lithosphere, Earth-science reviews. Wang, Z., Kusky, T. M., & Capitanio, F. A., 2017. Ancient continental lithosphere dislocated beneath ocean basins along the mid-lithosphere discontinuity: A hypothesis, Geophysical Research Letters, 44(18), 9253-9260. Yoshida, M., 2012. Dynamic role of the rheological contrast between cratonic and oceanic lithospheres in the longevity of cratonic lithosphere: A three-dimensional numerical study, Tectonophysics, 532, 156-166. Yoshida, M. & Yoshizawa, K., 2020. Continental drift with deep cratonic roots, Annual Review of Earth and Planetary Sciences, 49. Yuan, H. & Romanowicz, B., 2010. Lithospheric layering in the North American craton, Nature,466(7310), 1063-1068.