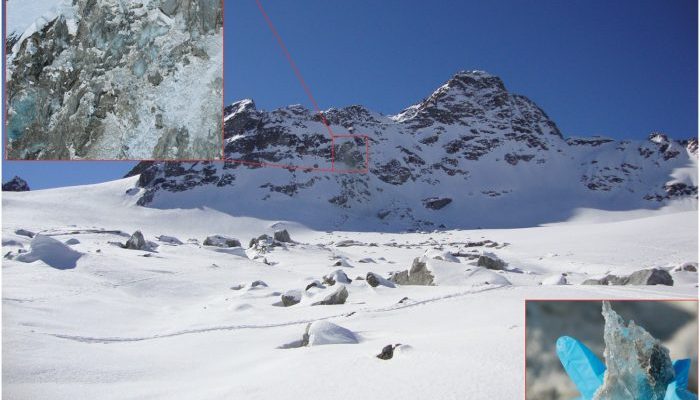
Rockfalls, rock slides and rock avalanches in high mountains
The terms rockfall, rock avalanche and rockslide are often used interchangeably. Different authors have proposed definitions based on volume thresholds, but the establishment of fixed boundaries can be tricky. Rockfall can be defined as the detachment of a mass of rock from a steep rock-wall, along discontinuities and/or through rock bridge breakage, and its free or bounding downslope movement under the influence of gravity[1,2]. Usually, we use this term when the volume is limited, and there is little dynamic interaction between rock fragments, which interact mainly with the substrate. Rockslides involve a larger volume (up to 100,000 m3) and the blocks often break in smaller fragments as they travel down the slope. In both rockfall and rockslide, the blocks move downslope mainly by falling, bouncing and rolling. On the other hand, rock avalanches involve the disintegration of rock fragments to form a downslope rapidly flowing, granular mass demonstrating exceptionally high mobility[3]. The size of these rock failures can vary from single boulders to several million cubic meters (e.g. the catastrophic failures of Triolet, 1717, and Randa, 1991).
Rock failures are widespread at any latitude and elevation, from mountain environments to coastal cliff, affecting the evolution and stability of different landscapes. They are among the most hazardous geomorphological processes, because of the velocity and the volume that these phenomena can involve. In mountainous areas, infrastructures, local populations and tourism can be directly threatened.
Rock slopes stability varies along time, especially in high mountains landscapes, in which the influencing external factors (e.g. hydrogeological conditions, and ice mass distribution) are changing during glacial cycles. Especially at high elevations, climatic factors are critical for rockfall stability (and others instability phenomena as rock avalanches or rock glaciers destabilisation), since they control weathering processes (mainly through temperature regime and precipitation activity) and because of rock-wall permafrost warming.
Rock instability triggering factors and the role of permafrost
The presence of damaged areas, weakness planes and discontinuities are essential to the development of instability. Focusing on high mountain environments, despite preconditioning factors as lithology and rock mass quality[4], the main preparatory and triggering factors have been recognized in: seismic activity[5,6], pressure release following glacier retreat[7], glacial erosion, including valley steepening, deepening and undercutting[4], and climate change, also involving permafrost degradation[8,9].
Permafrost is ground, both soil and rock, which has been frozen (temperature below 0°C) for two or more years[10]. Its climatically-driven degradation is considered nowadays one of the primary mechanisms in which climate is influencing slope stability[8]. It can involve ground ice temperature rising, ice-content modification or permafrost disappearance, especially at lower altitudes[11]. Several studies have highlighted the relationship between rockfall and permafrost degradation, especially in marginal permafrost conditions, albeit the level of dependence is still not clear and the role of other factors (e.g. rock mass quality) cannot be neglected. For instance, among the events occurred during the last century in the European Alps, the Brenva Glacier rock avalanches of 1920 and 1997 in the Aosta Valley (Italy), Cresta Guzza (Val Malenco, Italy) in 2013 (probably reported here for the first time, see details below and Figure 3), the recent instability in the Pizzo Cengalo, in 2012 and 2017, can be related to warm permafrost.
Glacier retreat and changes in permafrost, as related to present atmospheric warming, may destabilise over-steepened rock walls and accelerate cliff retreat in high mountain areas[12,13]. Ground ice melt, due to temperature increase and related changes in the groundwater regimes, influences the stability of rock walls located within the permafrost altitude belt, i.e. the altitude at which permafrost presence is confirmed[14,15]. Permafrost can affect rock walls stability through different processes, often acting together, as shear strength reduction due to interstitial ice melting, loss of ice-rock interlocking, increase in rock damage and fracture propagation due to freeze and thaw cycles or frost-cracking processes, changes in hydraulic conductivity and pore water pressure[16,17]. The presence of permafrost in the affected rock-walls is commonly evidenced by ice directly observable in more than a quarter of the rockfall scars analysed, see an example in Figure 1[13]. Climatic oscillations have a strong influence on all the processes mentioned above, as they are strictly related to temperature-driven ice dynamics and water availability.
In the last decades, an increased pattern of rockfall activity, on the entire Alpine arc has been observed[9]. At the same time, permafrost temperatures are showing an upward trend in the Alps[18], possibly leading to a growth of permafrost-related slope instabilities. Ravanel et al. (2017) explored the relation between increased temperatures and number of rockfalls in the Mount Blanc massif. The yearly number of rockfalls showed a strong increase (three to four times the average) in 2003 and 2015, years characterized by a summer heatwave.
Historical and recent events
One of the first recorded and documented event (Table 1) dates in 1717[19] at Triolet. During the night of 12-13 September, a massive block of ice-clad rock plunged onto the Triolet glacier from the crest of the towering Mount Blanc massif in northwestern Italy. Travellers accounts reported the event for more than a century after, due to the extensive damage and life losses it caused.
On 18th of September 2004 a rock avalanche of about 3 million m3 detached from the South-East flank of the Punta Thurwieser ridge (Italian Central Alps). Global warming has been recognised to have influenced this failure, namely through permafrost degradation and changes in glacier extension[20,21]. In this case, the runout was unusually long and rapid (Figure 2), mainly due to the propagation path, partly involving the Vedretta dello Zebrù glacier, and to the involved material, including rock, debris, ice and snow.
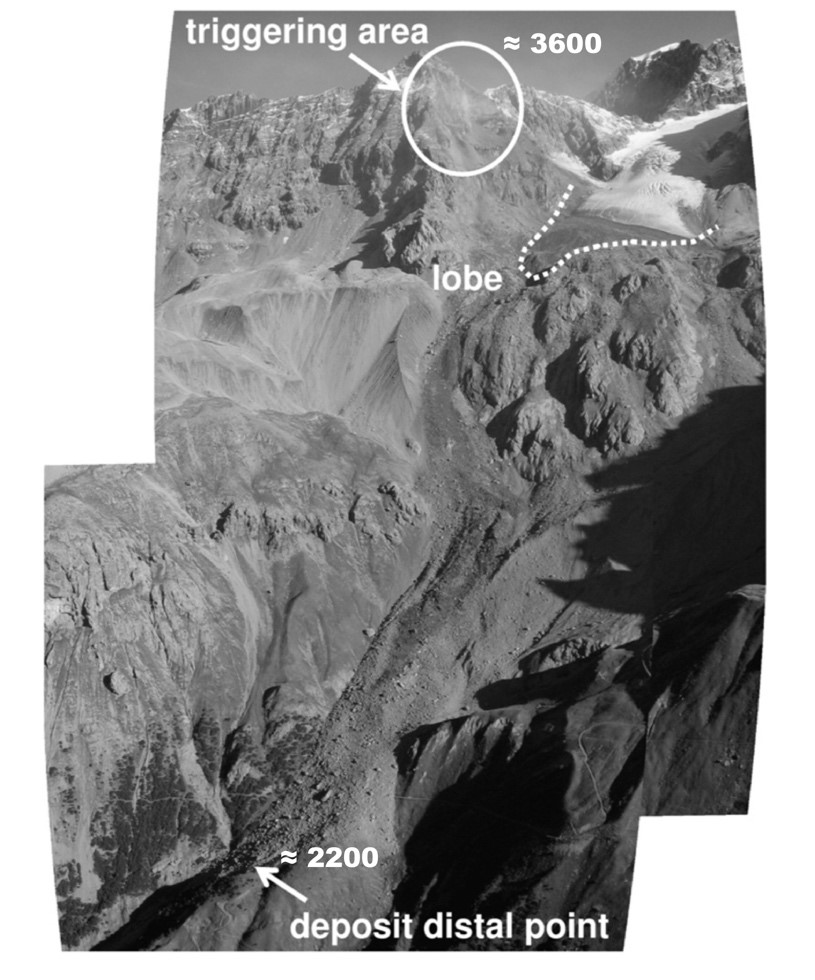
Figure 2 -the Punta Thurwieser rock avalanche from 2004 (modify after Pirulli, 2009[20])

Figure 3 –photo after the rockfall that involved Cresta Guzza in 2013 (photo courtesy of G. Neri and R. Scotti)
More recently, on 23rd August 2017, a rock failure, of about 4 million m3, occurred on the north face of Pizzo Cengalo (Figure 4), upper Val Bondasca (lateral valley of the Bregaglia Valley, on the Swiss Alps). The onset of the event and the subsequent rock avalanche was recorded on video. The rock avalanche then travelled on the Cengalo glacier, turning into a massive debris flow when it reached the Bondasca river. In 2012 already, a smaller rockfall event took place at Pizzo Cengalo and deposited 1.5 million m³ debris at the foot of the mountain.
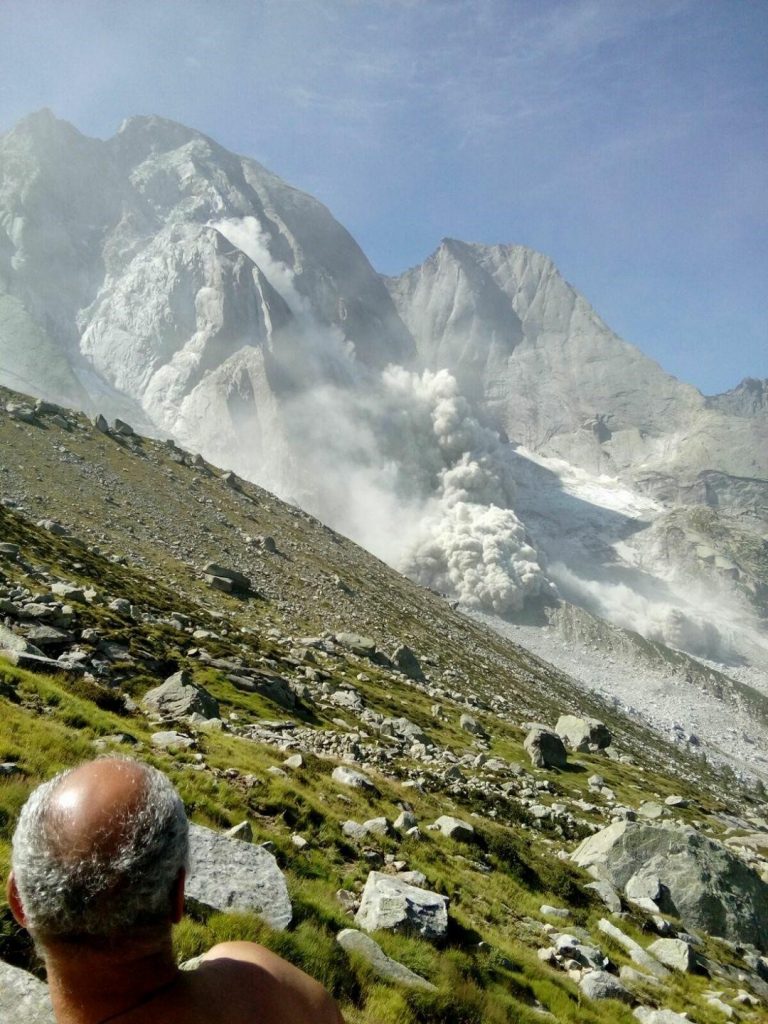
Figure 4 – a photo of the event that involved Pizzo Cengalo in 2017 (photo credit D. Chindam)
Finally, one of the last recorded events occurred at Flüela Wisshorn (Swiss Alps) on 18th March 2019 (Figure 5). A rockslide, involving about 250,000 m3 of material detached from the northwest flank of the mountain, video here. The detached rock travelled across a very famous ski tour route, but no one was injured, only thanks to the timing of the event, which occurred shortly after midnight. According to a preliminary photo interpretation, the failure surface suggests the presence of preexistent discontinuities and some breakage along the intact rock. However, more accurate investigations are still needed.
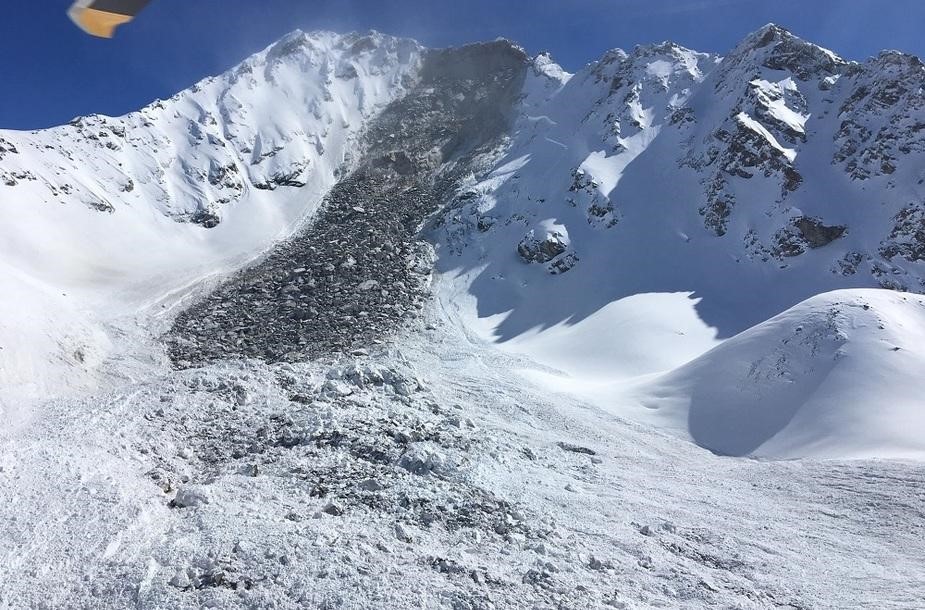
Figure 5 – photo after the rockslide event on Flüela Wisshorn (photo credit N. Conrad)
To conclude, we summarise the main known rockfall events along the Alps at high altitude in Table 1. However, as we said before many more events may have occurred that we are not aware of and thus we believe this list may not be complete. If someone is aware of other cases, we will be glad to add them! Just leave a comment below, and we will come back to you.
Table 1 – major rockfall event at high altitude reported on the Alps.
Name | Location | Date | Altitude [m asl] | Volume [106 m3] |
Triolet | I | 1717 | 3600 | 16-20 |
Fletschhorn | CH | 1901 | 3615 | 0.8 |
Brenva I | I | 1920 | 4200 | 2-3 |
Felik | I | 1936 | 3585 | 0.2 |
Jungfrau | CH | 1937 | 3800 | 0.15 |
Matterhorn | I | 1943 | 4150 | 0.24 |
Miage I | I | 1945 | 3050 | 0.3 |
Becca di Luseney | I | 1952 | 3150 | 11.0 |
Druesberg | CH | 1987 | 2100 | 0.07 |
Tschierva | CH | 1988 | 3280 | 0.3 |
Piz Scerscen | CH | 1988 | 3750 | – |
Randa | CH | 1991 | 2300 | 30 |
Miage II | I | 1991 | 3000 | 0.3 |
Zuetribistock | CH | 1996 | 2250 | 1.1 |
Brenva II | I | 1997 | 3725 | 2 |
Matternberg | CH | 1996 | 2720 | 0.1 |
Zugspitze | D | 2001 | 2630 | 0.03 |
Monte Rosa | I | 2001 | 3100 | 0.005 |
Gruben | CH | 2002 | 3520 | 0.1 |
Aiguille du Diable | FR | 2003 | 4114 | 0.020 |
Pointe du Domino | FR | 2003 | 3648 | 0.025 |
Thurwieser I | I | 2004 | 3658 | 2.5-3 |
Thurwieser II | I | 2010 | 3658 | 0.1 |
Pizzo Cengalo | CH | 2012 | ≈ 2500 | 1.5 |
Cresta Guzza | I | 2013 | ≈ 3600 | – |
Piz Kesch | CH | 2014 | ≈ 3400 | 0.15 |
Punta Tre Amici | I | 2015 | ≈ 3600 | 0.020 |
Aiguille du Tacul | FR | 2015 | 4248 | 0.016 |
Tour Ronde I | FR | 2015 | 3792 | 0.015 |
Tour Ronde II | FR | 2015 | 3792 | 0.002 |
Aiguille du Plan | FR | 2015 | 3673 | 0.012 |
Aiguille du Midi | FR | 2015 | 3842 | 0.012 |
Petit Dru – Bonatti | I | 2015 | ≈ 3700 | – |
Pizzo Cengalo | CH | 2017 | ≈ 2500 | 4 |
Flüela Wisshorn | CH | 2019 | ≈ 3000 | 0.25 |
About the Authors:
Stefano Alberti is a postdoc research fellow at Politecnico di Milano, Italy, and a landslide and geomechanics specialist. He obtained his PhD in Earth Sciences, curriculum in Engineering Geology, at Università degli Studi di Milano-Bicocca, Italy, in collaboration with the Kyoto University. Active collaborator of the Regional Glacial Survey of Lombardy (SGL). He is interested in the interplay among geological structure, the thermo-hydro-mechanical behaviour of rocks, slope instability processes on different spatial and temporal scales and their geomorphological, geohazards and engineering aspects. His research focuses on mechanisms and long-term evolution of rock slope deformation; monitoring and modelling of large landslides (e.g. MPM); laboratory rock deformation; geomorphological aspects in periglacial and glacial environments (e.g. rock glacier and permafrost distribution); rock fall modelling and protection.
Margherita Cecilia Spreafico is a postdoc researcher at the Università degli Studi di Milano-Bicocca, Italy, working on the long-term activity, damage and collapse potential of large slow-moving landslides in rock. She obtained her PhD in Civil, Environmental and Materials Engineering at the Università degli studi di Bologna, Italy, in collaboration with the Simon Fraser University in Vancouver, Canada. Her research interests focus on large landslides mechanisms, kinematics and dynamics; numerical modelling of complex landslides; factors predisposing and controlling slope failures; rock mechanics, including rock mass characterisation from terrestrial laser scanner and photogrammetric data; discrete fracture networks and rock bridges; the influence of the groundwater flow on slope stability
REFERENCES
- Deline, P., Jaillet, S., Rabatel, A., & Ravanel, L. (2008, June). Ground-Based LiDAR data on permafrost-related rock fall activity in the Mont-Blanc massif. In Proceedings of the 9th International Conference on Permafrost(pp. 349-354).
- Luckman, B. H. (2013). 7.17 Processes, Transport, Deposition, and Landforms: Rockfall.
- Hungr, O., Leroueil, S., & Picarelli, L. (2014). The Varnes classification of landslide types, an update. Landslides, 11(2), 167-194
- McColl, S. T. (2012). Paraglacial rock-slope stability. Geomorphology, 153, 1-16.
- Keefer, D. K. (2002). Investigating landslides caused by earthquakes–a historical review. Surveys in Geophysics, 23(6), 473-510.
- Jibson, R. W., Harp, E. L., Schulz, W., & Keefer, D. K. (2004). Landslides triggered by the 2002 Denali Fault, Alaska, earthquake and the inferred nature of the strong shaking. Earthquake Spectra, 20(3), 669-691.
- Ballantyne, C. K. (2002). Paraglacial geomorphology. Quaternary Science Reviews, 21(18-19), 1935-2017.
- Gruber, S., & Haeberli, W. (2007). Permafrost in steep bedrock slopes and its temperature‐related destabilization following climate change. Journal of Geophysical Research: Earth Surface, 112(F2).
- Ravanel, L., Magnin, F., & Deline, P. (2017). Impacts of the 2003 and 2015 summer heatwaves on permafrost-affected rock-walls in the Mont Blanc massif. Science of the Total Environment, 609, 132-143.
- Dobinski, W. (2011). Permafrost. Earth-Science Reviews, 108(3-4), 158-169.
- Marchenko, S. S., Gorbunov, A. P., & Romanovsky, V. E. (2007). Permafrost warming in the Tien Shan mountains, central Asia. Global and Planetary Change, 56(3-4), 311-327.
- Noetzli, J., Hoelzle, M., & Haeberli, W. (2003, July). Mountain permafrost and recent Alpine rock-fall events: a GIS-based approach to determine critical factors. In Proceedings of the 8th International Conference on Permafrost(Vol. 2, pp. 827-832). Balkema Zurich, Switzerland.
- Phillips, M., Wolter, A., Lüthi, R., Amann, F., Kenner, R., & Bühler, Y. (2017). Rock slope failure in a recently deglaciated permafrost rock wall at Piz Kesch (Eastern Swiss Alps), February 2014. Earth Surface Processes and Landforms, 42(3), 426-438.
- Dramis, F., Govi, M., Guglielmin, M., & Mortara, G. (1995). Mountain permafrost and slope instability in the Italian Alps: the Val Pola landslide. Permafrost and Periglacial Processes, 6(1), 73-81.
- Deline, P. (2001). Recent Brenva rock avalanches (Valley of Aosta): new chapter in an old story. Supplemento Geografia Fisica e Dinamica Quaternaria, 5, 55-63.
- Matsuoka, N., & Murton, J. (2008). Frost weathering: recent advances and future directions. Permafrost and Periglacial Processes, 19(2), 195-210.
- Krautblatter, M., Funk, D., & Günzel, F. K. (2013). Why permafrost rocks become unstable: a rock–ice‐mechanical model in time and space. Earth Surface Processes and Landforms, 38(8), 876-887.
- Mühll, D. V., Stucki, T., & Haeberli, W. (1998, June). Borehole temperatures in alpine permafrost: a ten year series. In Seventh International Conference(pp. 1089-1095).
- Porter, S. C., & Orombelli, G. (1981). Alpine Rockfall Hazards: Recognition and dating of rockfall deposits in the western Italian Alps lead to an understanding of the potential hazards of giant rockfalls in mountainous regions. American Scientist, 69(1), 67-75.
- Pirulli, M. (2009). The Thurwieser rock avalanche (Italian Alps): Description and dynamic analysis. Engineering Geology, 109(1-2), 80-92.
- Frattini, P., Riva, F., Crosta, G. B., Scotti, R., Greggio, L., Brardinoni, F., & Fusi, N. (2016). Rock-avalanche geomorphological and hydrological impact on an alpine watershed. Geomorphology, 262, 47-60.
Post edited by: Valeria Cigala and Gabriele Amato
Peter
I saw a rockfall about here on 21. July 2015 around 11:00: https://www.google.com/maps/place/47%C2%B033'28.5%22N+14%C2%B039'46.2%22E/@47.5579316,14.660637,1068m/data=!3m2!1e3!4b1!4m6!3m5!1s0x0:0xd7adc223da64de09!7e2!8m2!3d47.5579285!4d14.6628305!5m1!1e4
It must’ve been a small one, there was no dust and it settled in about 5 seconds. The rocks seemed to be the size of a small house.