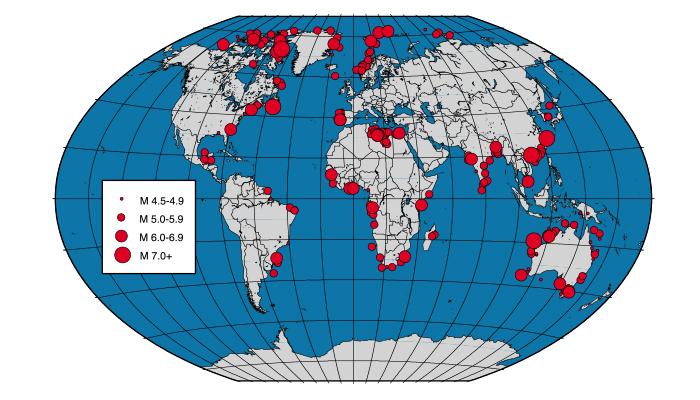
One of the basic tenets of plate tectonics states that deformation occurs along plate boundaries while plate interiors remain almost undeformed. Intraplate earthquakes defy this principle and hence are quite enigmatic. In this week’s News and Views, Prof. Attreyee Ghosh from the Centre for Earth Sciences, Indian Institute of Science, tries to explain the reasons behind intraplate earthquakes in central and eastern United States.
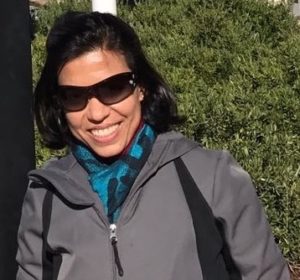
Prof. Attreyee Ghosh from Centre for Earth Sciences, Indian Institute of Science
Most major earthquakes are associated with plate boundaries (Figure 1). The 2004 Indian Ocean earthquake as well as the 2011 Tohuku earthquake, both of which produced large tsunamis were subduction zone earthquakes, where a cold lithospheric slab is diving into the mantle. The largest earthquake recorded so far, the 1960 Chile earthquake, also occurred in a subduction setting. The western boundary of the North American continent, the location of the transform San Andreas fault, has also seen its fair share of major earthquakes. The collisional boundary between India and Eurasia is seismically very active and has experienced destructive earthquakes in the past. The reason behind these earthquakes is the grinding motion of two plates as they collide with each other or slide past each other. However, although rare, earthquakes do occur within the plate interiors, defying one of the basic tenets of plate tectonics that states that all deformation takes place along the plate boundaries, whereas the plate interiors are undeformed. In fact, one of the most destructive earthquakes of all time have occurred in the middle of North America, in a place called New Madrid (Figure 2). In the years 1811-1812 a sequence of major earthquakes (magnitude 8) shook the New Madrid region. The 1886 Charleston earthquake, with an estimated magnitude of approximately 7 as well as the more recent Mineral Virginia earthquake of 2011, show that the North American plate is not as seismically inactive as we think it is. Elsewhere in the world, a magnitude 7.7 earthquake occurred in 2001 within the Indian plate at a town called Bhuj. All these along with several other events (Schulte & Mooney, 2005) indicate that large earthquakes do occur within plate interiors. The question is, how? A fundamental understanding of these intraplate earthquakes, which is crucial for a better seismic hazard assessment, is still elusive.
It is generally agreed that these intraplate earthquakes are caused by reactivation of old rifts (Schulte & Mooney, 2005; Stein et al., 1989; Sykes, 1978; Zoback & Richardson, 1996). However, what causes this reactivation is not well-understood. The intraplate earthquakes of North America have been attributed to various factors, such as glacio-isostatic adjustment (GIA, Mazzotti et al., 2005; Muir-Wood, 2000), weakening of mantle due to rifting or arrival of a plume (Chen et al., 2016), ridge push (Zoback & Zoback, 1981, 1980), gravitational body forces (Levandowski et al., 2017, 2017), large scale mantle convection (Forte et al., 2007) as well as dynamic topography (Becker et al., 2015; Forte et al., 2010). In a recent study (Ghosh et al., 2019), we evaluated the role of large scale tectonic forces in reactivating pre-existing faults and causing earthquakes within the North American plate interior. We quantified the effective body forces arising from lithosphere structure and topography coupled with global mantle flow. We predicted stress and strain rate patterns and compared them with seismic and geodetic observations, such as earthquake P-axes, moment tensors and earthquake-inversion stresses. We also tested the role of first order lateral viscosity variations.
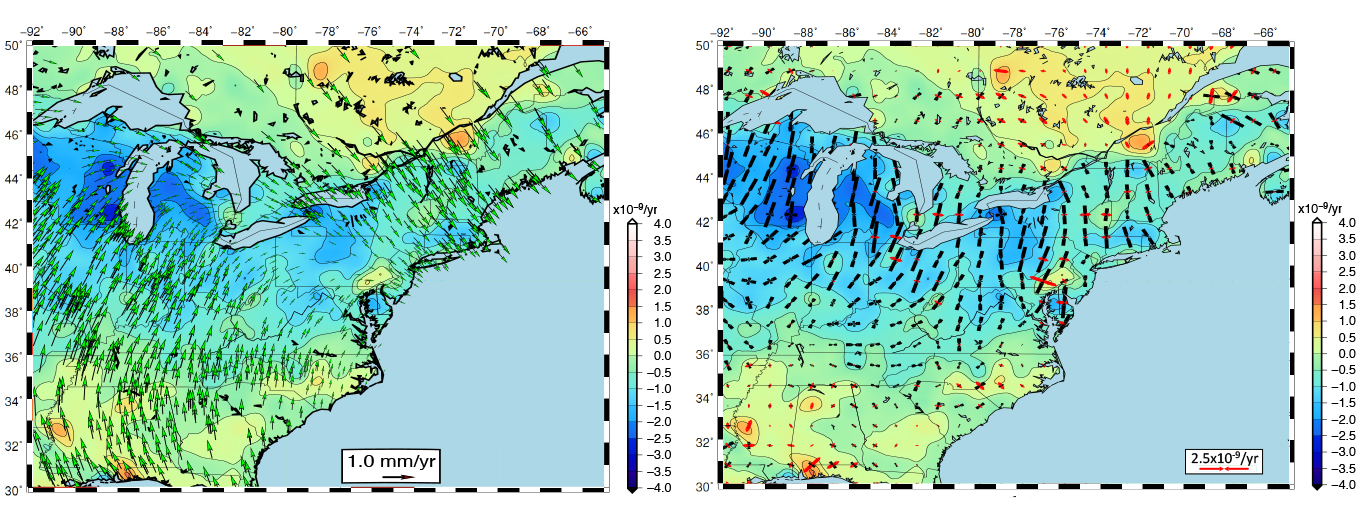
Fig 3: (left) Model velocity field fit to observed GPS field. (right) Principal axes of strain rates derived from the velocity field plotted on top of dilatational strain rates. Figures published in Ghosh et al. 2019.
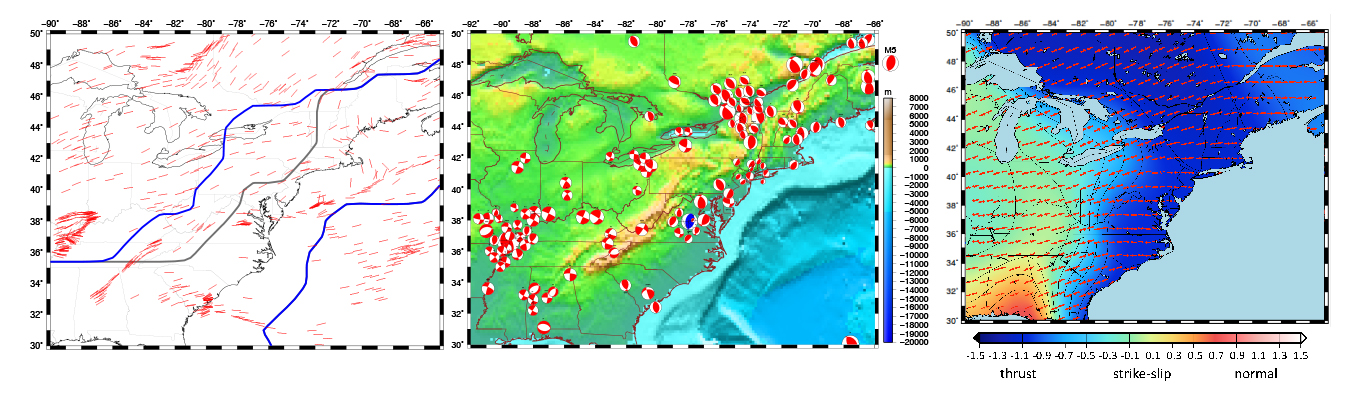
Fig 4: (left) Stress inversions of Levandowski et al. (2018). Blue lines mark the eastern margin of the craton and the western margin of the strong west Atlantic block, whereas the gray line delineates the Appalachian gravity gradient boundary. (middle) Focal mechanisms of eastern U.S. earthquakes compiled from various sources plotted on topography. (right) Directions of maximum shortening (red principal axes) obtained from moment tensors of all the earthquakes plotted on focal mechanism style. Figures published in Ghosh et al. 2019.
What we found is that while the GIA predicted strain rate pattern are oriented radially outward from the paleo ice load (Figure 3), the orientation of the P-axes as well as the inverted stress directions from earthquakes are NE-SW to E-W oriented (Figure 4). This could rule out the contribution of GIA in causing the North American intraplate earthquakes.
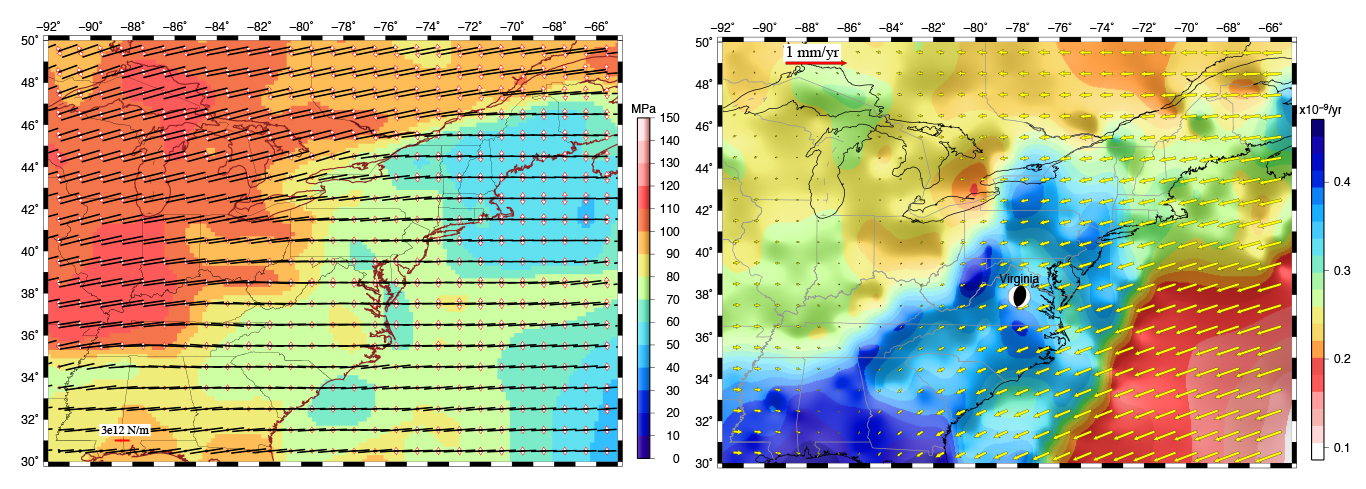
Fig 5: (left) Predicted deviatoric stresses from our best model plotted on top of their second invariants. Black arrows indicate compression, whereas red indicate tensional stresses. (right) Relative velocities (yellow vectors) plotted on top of predicted strain rates from our best model. Figures published in Ghosh et al. 2019.
Our predicted tectonic stresses, on the other hand, have the SHmax axes (most compressive stress direction) oriented in the same direction as the P-axes of earthquakes, moment tensors and stress inversions (Figure 5, left panel). We also found that lateral viscosity variations within the lithosphere have a role to play in affecting the stress pattern, with a viscosity structure comprising of strong cratons and oceanic lithosphere (approximately 1025 Pas), moderately strong intraplate areas (5X1024 Pas) and weak plate boundaries (1021-1023 Pas) yielding the best results. This particular model predicts strain rate magnitudes of 3-5X10-10/year (Figure 5, right panel), which are within the acceptable range of long term strain rates inferred from GPS studies.
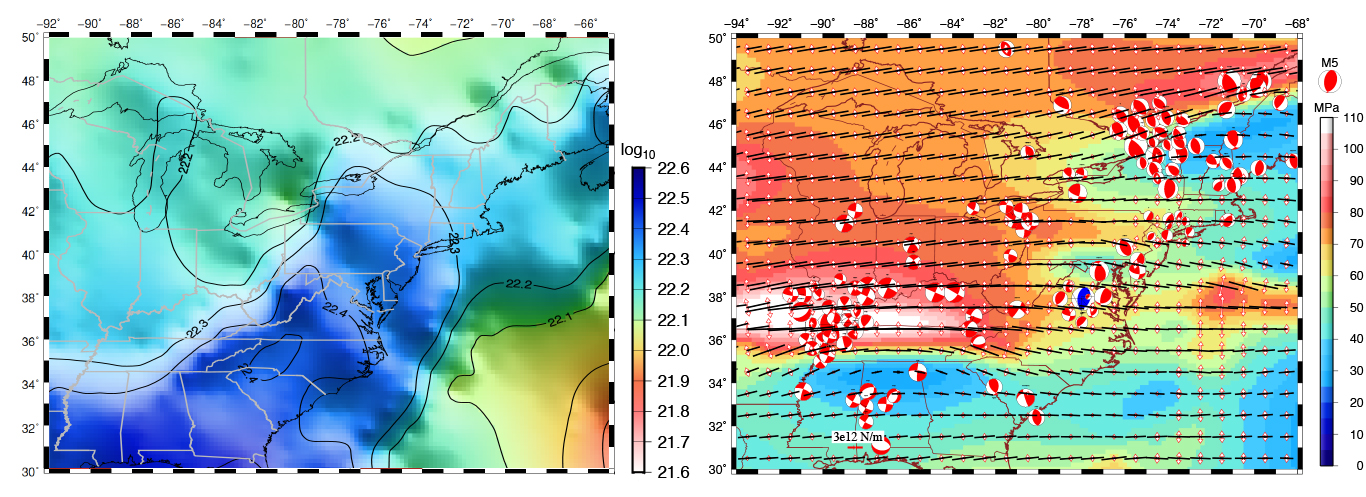
Fig 6: (left) Tectonic moment rates in dyne-cm/year predicted from our best model. (right) Predicted deviatoric stresses plotted on their magnitudes (second invariants) from a model with high viscosity contrast between craton and the weaker zone of accreted Appalachian terranes. Observe the high stresses within the New Madrid region. Figures published in Ghosh et al. 2019.
These long term strain rates, when converted to seismic moment rates (Holt et al., 1995, 2000), translate to a moment (Figure 6) that is equivalent to about three magnitude 7.1 earthquakes over a 300 year period in the central and eastern US region. We also observed a focusing of stress within the New Madrid region, which arises due to the strength contrast between the strong cratonic interior and the weaker Appalachian terrane (Figure 6). This strength contrast could be a potential reason for high seismicity in the area.
Overall, we conclude that long wavelength tectonic stresses, arising from plate boundary forces, can match the earthquake stress and strain indicators in central and eastern US and could be responsible for the intraplate earthquakes in this region. Our model only consists of first order strength variations. Further refinements in sublithospheric density contrasts as well as lateral strength contrasts, can provide more accurate estimates of long term deformation and seismic hazard in this region.
Reference: Becker, T. W., Lowry, A. R., Faccenna, C., Schmandt, B., Borsa, A., & Yu, C. (2015). Western US intermountain seismicity caused by changes in upper mantle flow. Nature, 524, 458–461. Chen, C., Gilbert, H., Andronicos, C., Hamburger, M. W., Larson, T., Marshak, S., et al. (2016). Shear velocity structure beneath the central United States: Implications for the origin of the Illinois Basin and intraplate seismicity. Geochemistry, Geophysics, Geosystems, 17, 1020–1041. https://doi.org/10.1002/2015GC006206 Forte, A. M., Mitrovica, J. X., Moucha, R., Simmons, N. A., & Grand, S. P. (2007). Descent of the ancient Farallon slab drives localized mantle flow below the New Madrid seismic zone. Geophysical Research Letters, 34, L04308. https://doi.org/10.1029/2006GL027895 Forte, A., Moucha, R., Simmons, N., Grand, S., & Mitrovica, J. (2010). Deep-mantle contributions to the surface dynamics of the North American continent. Tectonophysics, 481, 3–15. https://doi.org/10.1016/j.tecto.2009.06.010 Ghosh, A., Holt, W. E., & Bahadori, A. (2019). Role of large‐scale tectonic forces in intraplate earthquakes of Central and Eastern North America. Geochemistry, Geophysics, Geosystems, 20(4), 2134-2156. Mazzotti, S., James, T. S., Henton, J., & Adams, J. (2005). GPS crustal strain, postglacial rebound, and seismic hazard in eastern North America: The Saint Lawrence valley example. Journal of Geophysical Research, 110, B11301. https://doi.org/10.1029/2004JB003590 Mazzotti, S., & Townend, J. (2010). State of stress in central and eastern North American seismic zones. Lithosphere, 2, 76–83. https://doi. org/10.1130/L65.1 Muir-Wood, R. (2000). Deglaciation seismotectonics: A principal influence on intraplate seismogenesis at high latitudes. Quaternary Science Reviews, 19, 1399–1411. Schulte, S., & Mooney, W. D. (2005). An updated global earthquake catalogue for stable continental regions: Reassessing the correlation with ancient rifts. Geophysical Journal International, 161, 707–721. Stein, S., Cloetingh, S., Sleep, N. H., & Wortel, R. (1989). Passive margin earthquakes, stresses and rheology. In S. Gregerson, & P. Basham (Eds.), Earthquakes at North-Atlantic passive margins: Neotectonics and postglacial rebound (pp. 231–260). Dordecht: Kluwer. Sykes, L. (1978). Intraplate seismicity, reactivation of preexisting zones of weakness, alkaline magmatism, and other tectonism postdating continental fragmentation. Reviews of Geophysics, 16, 621–688. Wolin, Emily, Seth Stein, Frank Pazzaglia, Anne Meltzer, Alan Kafka, and Claudio Berti. "Mineral, Virginia, earthquake illustrates seismicity of a passive‐aggressive margin." Geophysical Research Letters 39, no. 2 (2012). Zoback, M. L., & Richardson, R. M. (1996). Stress perturbation associated with the Amazonas and other ancient continental rifts. Journal of Geophysical Research, 101, 5459–5475. Zoback, M. L., & Zoback, M. D. (1980). State of stress in the conterminous United States. Journal of Geophysical Research, 85, 6113–6156. Zoback, M. D., & Zoback, M. L. (1981). State of stress and intraplate earthquakes in the United States. Science, 213, 96–104.