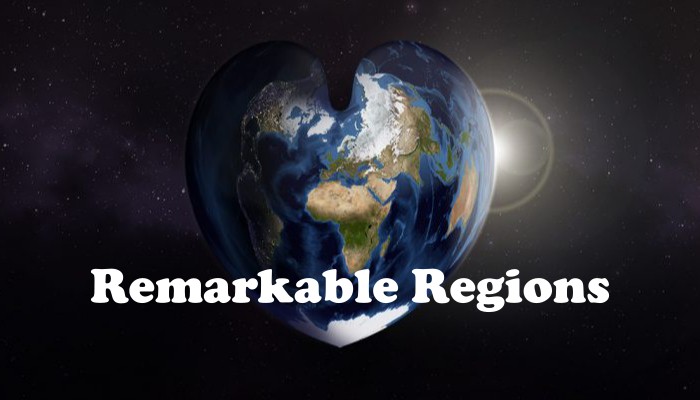
Every 8 weeks we turn our attention to a Remarkable Region that deserves a spot in the scientific limelight. After exploring the Mediterranean and the ancient Tethys realm, we now move further north and across the Pacific to the Aleutian-Alaska subduction zone. This post was contributed by Kirstie Haynie who is a PhD candidate at the department of geology at the University at Buffalo, State University of New York, in the United States of America.
Given that Alaska is a remarkable region, I decided to walk up to strangers and ask them what comes to mind when they hear the word “Alaska”. Indeed I received some confusing looks and laughs, but everyone I asked had something to say. Some people alluded to popular TV shows set in Alaska, such as Gold Rush, Bush People, and Alaska: the Last Frontier, while others spoke about the cold weather, dog mushing, Eskimos, fishing and hunting, and the Trans-Alaska pipeline. A few of the answers I received referenced the beauty and wilderness of the large snow capped mountains, glaciers, and the Northern Lights (Aurora Borealis): all emblematic of the largest state in America. But to me, Alaska is more than just a pretty landscape and a place to fish. It is a region riddled with geologic mysteries and rich in along strike variations.
The Aleutian-Alaska subducton zone marks a North American-Pacific plate boundary where subduction varies greatly along strike (Figure 1). At the western end of the subduction zone, the Aleutian volcanic islands are the result of oceanic-oceanic subduction while in the eastern part of the subduction zone there is oceanic-continental collision where the Pacific plate descends beneath the North American plate. The age of the subducting sea floor increases laterally from around 30 Ma in the eastern subduction corner to 80 Ma at the end of the Aleutian volcanic arc (Müller et al., 2008). Slab dip changes drastically from 50° to 60° in the west and central Aleutians to flat slab subduction under south-central Alaska (Ratchkovski and Hansen, 2002a; Lallemand et al., 2005; Jadamec and Billen, 2010). This leads to a variation in the slab pull force, which is a main driving force of subduction caused by the weight of dense slabs sinking into the mantle (Morra et al., 2006).
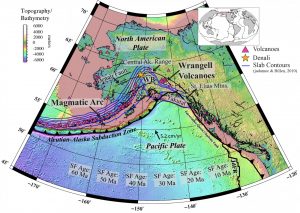
Figure 1: Tectonic map of Alaska modified from Haynie and Jadamec (2017). Topography/bathymetry is from Smith and Sandwell (1997) and Seafloor (SF) ages are from Müller et al. (2008). Blue lines are the slab contours of Jadamec and Billen (2010) in 40 km intervals; the thick black line is the plate boundary from Bird (2003); and the thinner black lines are faults from Plafker et al. (1994a). The location of Denali is marked by the orange hexagon. Holocene volcanoes are given by the pink triangles (Alaska Volcano Observatory). The purple polygon is the outline of the Yakutat oceanic plateau (Haynie and Jadamec, 2017). WB – Wrangell block fore-arc sliver; JdFR – Juan de Fuca Ridge.
There is also a distinct change in margin curvature from convex in the west to concave in the east. At the end of the eastern bend, the Alaska part of the subduction zone is truncated by a large transform boundary, the Fairweather-Queen Charolette fault, which gives rise to a corner-shaped subduction-transform plate boundary (Jadamec et al., 2013; Haynie and Jadamec, 2017). Here, convergence is oblique with an average velocity of 5.2 cm/year northwest (DeMets and Dixon, 1999). Seismic studies (Page et al., 1989; Ferris et al., 2003; Eberhart-Phillips et al., 2006; Fuis et al., 2008) show that thicker than normal oceanic crust lies off-shore in the subduction corner. This thick oceanic material has been identified as the Yakutat oceanic plateau (Plafker et al., 1994a; Brocher et al., 1994; Bruns, 1983; Worthington et al., 2008; Christeson et al., 2010; Worthington et al., 2012). Even though oceanic plateaus tend to resist subduction (Cloos, 1993; Kerr , 2003), the Yakutat plateau is currently subducting beneath the Central Alaska Range to depths of 150 km (Ferris et al., 2003; Eberhart-Phillips et al., 2006; Wang and Tape, 2014). It is also colliding into south-east Alaska (Mazzotti and Hyndman, 2002; Elliott et al., 2013; Marechal et al., 2015) where the largest coastal mountain range on Earth, the Saint Elias Mountains, are located (Enkelmann et al., 2015).
With regards to surface deformation, in addition to Denali (the tallest mountain in North America), other notable along strike variations reside within the broad deformation zone of south-central Alaska. For example, a normal volcanic arc occurs over the Aleutian part of the subduction zone and above the Alaska Peninsula. However, above the flat slab there is a gap in volcanism followed by the presence of the enigmatic Wrangell volcanoes (Rondenay et al., 2010; Jadamec and Billen, 2012; Martin-Short et al., 2016; Chuang et al., 2017). These volcanoes are marked by a range of morphologies as well as adakitic geochemical signatures (Richter et al., 1990; Preece and Hart , 2004), which have a petrogenesis that may be attributed to slab melting (Defant and Drummond , 1990; Peacock et al., 1994; Castillo, 2006, 2012; Ribeiro et al., 2016). Analogue (Schellart , 2004; Strak and Schellart , 2014) and 3D numerical models (Stegman et al., 2006; Piromallo et al., 2006; Jadamec and Billen, 2010, 2012) predict that toroidal flow can produce upwellings around the edge of a slab that may have implications for melting of the slab and the formation of adakites. However, the formation of the Wrangell volcanoes is still debated.
Also located above the subducting plateau and flat slab is the Wrangell block fore-arc sliver, which exhibits northwest motion and counterclockwise rotation (Cross and Freymueller, 2008; Freymueller et al., 2008; Bemis et al., 2015; Waldien et al., 2015; Jadamec et al., 2013; Haynie and Jadamec, 2017). This sliver is bounded in the north by the arcuate shaped Denali fault, which illustrates a lateral change in slip rates that increases towards the center of the fault (Haynie and Jadamec, 2017; Haeussler et al., 2017). 3D high-resolution geodynamic models show that the flat slab drives motion of the Wrangell block fore-arc sliver (Jadamec et al., 2013; Haynie and Jadamec, 2017) and contributes to fault parallel motion along the eastern Denali fault and convergence along the apex of the fault (Haynie and Jadamec, 2017) (Figure 2). However, when model predictions of the Wrangell block motion and the difference in Denali fault parallel motion are compared with observations, model predictions are lower, suggesting that the flat slab alone is not sufficient enough to explain the broad deformation zone of Alaska (Haynie and Jadamec, 2017). Thus, it is thought that the neotectonics of south-central Alaska are predominantly driven by the subduction-collision of the buoyant Yakutat oceanic plateau (Bird , 1988; Plafker et al., 1994b; Fitzgerald et al., 1995; Ratchkovski and Hansen, 2002b; Bemis and Wallace, 2007; Chapman et al., 2008; Haeussler , 2008; Jadamec et al., 2013; Lease et al., 2016; Haynie and Jadamec, 2017). 4D numerical modelling of this process is currently underway.
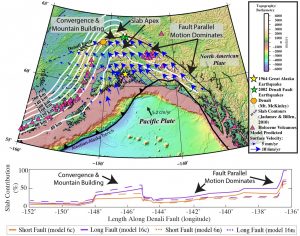
Figure 2: Top: map of south-central Alaska (zoomed in from Figure 1) with model predicted velocities (blue arrows) from Haynie and Jadamec (2017) plotted on top. Bottom: percent of slab contribution from Haynie and Jadamec (2017) models to observed Denali fault slip rates (modified from Haynie and Jadamec (2017)). Results from Haynie and Jadamec (2017) show that the slab drives northwest and counter-clockwise motion of the Wrangell block fore-arc sliver and contributes to an average of 20-28% of motion along the Denali fault. The flat slab exerts the largest contribution to motion along the eastern segment of the fault, where surface motion parallels the fault, and also along the central segment of the fault, where the slab is driving the Wrangell block into the North American backstop and subducting obliquely to the fault.
References Bemis, S. P., and W. K. Wallace (2007), Neotectonic framework of the north-central Alaska Range foothills, Geological Society of America Special Papers, 431, 549–572. Bemis, S. P., R. J. Weldon, and G. A. Carver (2015), Slip partitioning along a continuously curved fault: Quaternary geologic controls on Denali fault system slip partitioning, growth of the Alaska Range, and the tectonics of south-central Alaska, Lithosphere, 7 (3), 235–246. Bird, P. (1988), Formation of the Rocky Mountains, Western United States: A continuum computer model, Science, 239 (4847), 1501–1507. Bird, P. (2003), An updated digital model of plate boundaries, Geochemistry, Geophysics, Geosystems, 4 (3). Brocher, T. M., G. S. Fuis, M. A. Fisher, G. Plafker, Moses, M. J., J. J. Taber, and N. I. Christensen (1994), Mapping the megathrust beneath the northern gulf of alaska using wideangle seismic data, Journal of Geophysical Research: Solid Earth, 99 (B6), 11,663– 11,985. Bruns, T. R. (1983), Model for the origin of the Yakutat block, an accreting terrane in the northern Gulf of Alaska, Geology, 11 (12), 718–721. Castillo, P. R. (2006), An overview of adakite petrogenesis, Chinese Science Bulletin, 51 (3), 257–268. Castillo, P. R. (2012), Adakite petrogenesis, Lithos, 134, 304–316. Chapman, J. B., T. L. Pavlis, S. Gulick, A. Berger, L. Lowe, J. Spotila, R. Bruhn, M. Vorkink, P. Koons, A. Barker, et al. (2008), Neotectonics of the Yakutat collision: Changes in defor- mation driven by mass redistribution, Active Tectonics and Seismic Potential of Alaska, Geophys. Monogr. Ser, 179, 65–81. Christeson, G. L., H. J. A. Gulick, P. S. ad Van Avendonk, L. L. Worthington, R. S. Reece, and T. L. Pavlis (2010), The Yakutat terrane: Dramatic change in crustal thickness across the Transition fault, Alaska, Geology, 38 (10), 895–898. Chuang, L., M. Bostock, A. Wech, and A. Plourde (2017), Plateau subduction, intraslab seismicity, and the Denali (Alaska) volcanic gap, Geology, pp. G38,867–1. Cloos, M. (1993), Lithospheric bouyancy and collisional orogenesis: Subduction of oceanic plateaus, continental margins, island arcs, spreading ridges, and seamounts, Geological Society of America Bulletin, 105 (715-737). Cross, R. S., and J. T. Freymueller (2008), Plate coupling variation and block translation in the Andreanof segment of the Aleutian arc determined by subduction zone modeling using GPS data, Geophysical Research Letters, 34 (6). Defant, M. J., and M. S. Drummond (1990), Derivation of some modern arc magmas by melting of young subducted lithosphere, Nature, 347 (6294), 662–665. DeMets, C., and T. H. Dixon (1999), New kinematic models for Pacific-North America motion from 3 Ma to present, I: Evidence for steady motion and biases in the NUVEL-1A Model, Geophysical Research Letters, 26 (13), 1921–1924. Eberhart-Phillips, D., D. H. Christensen, T. M. Brocher, R. Hansen, N. A. Ruppert, P. J. Haeussler, and G. A. Abers (2006), Imaging the transition from Aleutian subduction to Yakutat collision in central Alaska, with local earthquakes and active source data, Journal of Geophysical Research: Solid Earth, 111 (B11). Elliott, J., J. T. Freymueller, and C. F. Larsen (2013), Active tectonics of the St. Elias orogen, Alaska, observed with GPS measurements, Journal of Geophysical Research: Solid Earth, 118 (10), 5625–5642. Enkelmann, E., P. O. Koons, T. L. Pavlis, B. Hallet, A. Barker, J. Elliott, J. I. Garver, S. P. Gulick, R. M. Headley, G. L. Pavlis, et al. (2015), Cooperation among tectonic and surface processes in the St. Elias Range, Earth’s highest coastal mountains, Geophysical Research Letters, 42 (14), 5838–5846. Ferris, A., G. A. Abers, D. H. Christensen, and E. Veenstra (2003), High resolution image of the subducted Pacific (?) plate beneath central Alaska, 50–150 km depth, Earth and Planetary Science Letters, 214 (3), 575–588. Fitzgerald, P. G., R. B. Sorkhabi, T. F. Redfield, and E. Stump (1995), Uplift and denuda- tion of the central Alaska Range: A case study in the use of apatite fission track ther- mochronology to determine absolute uplift parameters, Journal of Geophysical Research: Solid Earth, 100 (B10), 20,175–20,191. Freymueller, J. T., H. Woodard, S. C. Cohen, R. Cross, J. Elliott, C. F. Larsen, S. Hreins- dottir, and C. Zweck (2008), Active deformation processes in Alaska, based on 15 years of GPS measurements, Active tectonics and seismic potential of Alaska, 179, 1–42. Fuis, G. S., T. E. Moore, G. Plafker, T. M. Brocher, M. A. Fisher, W. D. Mooney, W. Nodle- berg, R. Page, B. Beaudoin, N. I. Christensen, A. Levander, W. Lutter, R. Saltus, and N. A. Ruppert (2008), Trans-Alaska Crustal Transect and continental evolution involving subduction underplating and synchronous foreland thrusting, Geology, 36 (3), 267–270. Haeussler, P. J. (2008), An Overview of the Neotectonics of Interior Alaska: Far-Field Defor- mation From the Yakutat Microplate Collision, American Geophysical Union, pp. 86–108. Haeussler, P. J., A. Matmon, D. P. Schwartz, and G. G. Seitz (2017), Neotectonics of interior alaska and the late quaternary slip rate along the denali fault system, Geosphere. Haynie, K., and M. A. Jadamec (2017), Tectonic drivers of the Wrangell block: Insights on forearc sliver processes from 3D geodynamic models of Alaska, Tectonics, 36 (7), 1180– 1206. Jadamec, M., and M. Billen (2010), Reconciling surface plate motions with rapid three- dimensional mantle flow around a slab edge, Nature, 465 (7296), 338–341. Jadamec, M. A., and M. I. Billen (2012), The role of rheology and slab shape on rapid mantle flow: Three-dimensional numerical models of the Alaska slab edge, Journal of Geophysical Research: Solid Earth, 117 (B2). Jadamec, M. A., M. I. Billen, and S. M. Roeske (2013), Three-dimensional numerical models of flat slab subduction and the Denali fault driving deformation in south-central Alaska, Earth and Planetary Science Letters, 376, 29–42. Kerr, A. C. (2003), Oceanic plateaus, The Crust, Treaste on Geochemistry, 3, 537–565. Lallemand, S., A. Heuret, and D. Boutelier (2005), On the relationships between slab dip, back-arc stress, upper plate absolute motion, and crustal nature in subduction zones, Geochemistry Geophysics Geosystems, 6 (9). Lease, R. O., P. J. Haeussler, and P. O'Sullivan (2016), Changing exhumation patterns during Cenozoic growth and glaciation of the Alaska Range: Insight from detrital geo-and thermo-chronology, Tectonics. Marechal, A., S. Mazzotti, J. L. Elliot, J. T. Freymueller, and M. Schmidt (2015), Indentor- corner tectonics in the Yakutat-St. Elias collision constrained by GPS, Journal of Geo- physical Research: Solid Earth. Martin-Short, R., R. M. Allen, and I. D. Bastow (2016), Subduction geometry beneath south-central Alaska and its relationship to volcanism, Geophysical Research Letters, doi: 10.1002/2016GL070580, 2016GL070580. Mazzotti, S., and R. Hyndman (2002), Yakutat collision and strain transfer across the north- ern Canadian Cordillera, Geology, 30 (6), 495–498. Morra, G., K. Regenauer-Lieb, and D. Giardini (2006), Curvature of oceanic arcs, Geology, 34 (10), 877–880. Müller, R. D., M. Sdrolias, C. Gaina, and W. R. Roest (2008), Age, spreading rates, and spreading asymmetry of the world’s ocean crust, Geochemistry, Geophysics, Geosystems, 9 (4). Page, R., C. Stephens, and J. Lahr (1989), Seismicity of the Wrangell and Aleutian Wadati- Benioff zones and the north American plate along the Trans-Alaska Crustal Transect, Chugach Mountains and Copper River Basin, Southern Alaska, Journal of Geophysical Research, 94 (B11), 16,059–16,082. Peacock, S. M., T. Rushmer, and A. B. Thompson (1994), Partial melting of subducting oceanic crust, Earth and planetary science letters, 121 (1), 227–244. Piromallo, C., T. Becker, F. Funiciello, and C. Faccenna (2006), Three-dimensional instan- taneous mantle flow induced by subduction, Geophysical Research Letters, 33 (8). Plafker, G., J. C. Moore, and G. R. Winkler (1994a), Geology of the southern Alaska margin, The Geology of North America, The Geology of Alaska, G-1. Plafker, G., L. M. Gilpin, and J. C. Lahr (1994b), Neotectonic map of Alaska, The Geology of North America, 1. Preece, S. J., and W. K. Hart (2004), Geochemical variations in the <5 Ma Wrangell Volcanic Field, Alaska: implications for the magmatic and tectonic development of a complex continental arc system, Tectonophysics, 392 (1), 165–191. Ratchkovski, N., and R. Hansen (2002a), New evidence for segmentation of the Alaska subduction zone, Bulletin Of The Seismological Society Of America, 92 (5), 1754–1765. Ratchkovski, N. A., and R. A. Hansen (2002b), New Constraints on Tectonics of Interior Alaska: Earthquake Locations, Source Mechanisms, and Stress Regime, Bulletin Of The Seismological Society Of America, 92 (3), 998–1014. Ribeiro, J. M., R. C. Maury, and M. Gr´egoire (2016), Are Adakites Slab Melts or High-pressure Fractionated Mantle Melts?, Journal of Petrology, 57 (5), 839, doi: 10.1093/petrology/egw023. Richter, D., J. G. Smith, M. Lanphere, G. Dalrymple, B. Reed, and N. Shew (1990), Age and progression of volcanism, wrangell volcanic field, alaska, Bulletin of Volcanology, 53 (1), 29–44. Rondenay, S., L. G. Mont´esi, and G. A. Abers (2010), New geophysical insight into the origin of the Denali volcanic gap, Geophysical Journal International, 182 (2), 613–630. Schellart, W. (2004), Kinematics of subduction and subduction-induced flow in the upper mantle, Journal of Geophysical Research: Solid Earth, 109 (B7). Smith, W. H., and D. T. Sandwell (1997), Global sea floor topography from satellite altimetry and ship depth soundings, Science, 277 (5334), 1956–1962. Stegman, D., J. Freeman, W. Schellart, L. Moresi, and D. May (2006), Influence of trench width on subduction hinge retreat rates in 3-D models of slab rollback, Geochemistry, Geophysics, Geosystems, 7 (3). Strak, V., and W. P. Schellart (2014), Evolution of 3-D subduction-induced mantle flow around lateral slab edges in analogue models of free subduction analysed by stereoscopic particle image velocimetry technique, Earth and Planetary Science Letters, 403, 368–379. Waldien, T., S. M. Roeske, J. A. Benowitz, W. K. Allen, and K. D. Ridgway (2015), Neogene exhumation in the eastern Alaska Range and its relationship to splay fault activity in the Denali fault system, AGU Fall Meeting Abstracts. Wang, Y., and C. Tape (2014), Seismic velocity structure and ansotropy of the Alaska subduction zone based on surface wave tomography, Journal of Geophysical Research: Solid Earth, 119, 8845–8865. Worthington, L. L., S. P. Gulick, and T. L. Pavlis (2008), Identifying active structures in the Kayak Island and Pamplona zones: Implications for offshore tectonics of the Yakutat Microplate, Gulf of Alaska, Active tectonics and seismic potential of Alaska: American Geophysical Union Geophysical Monograph, 179, 257–268. Worthington, L. L., H. Van Avendonk, S. Gulick, G. L. Christeson, and T. L. Pavlis (2012), Crustal structure of the Yakutat terrane and the evolution of subduction and collision in southern Alaska, Journal of Geophysical Research: Solid Earth, 117 (B1).