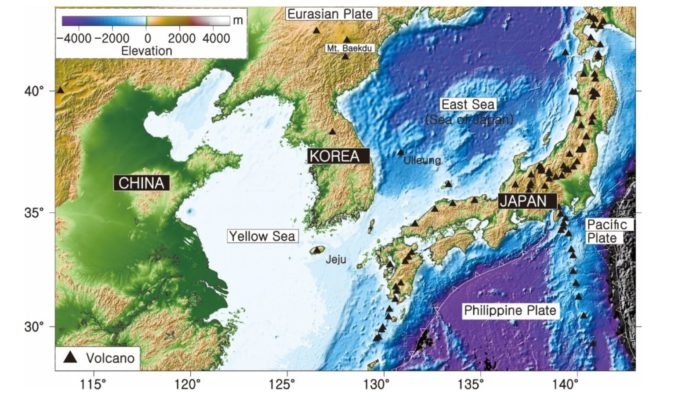
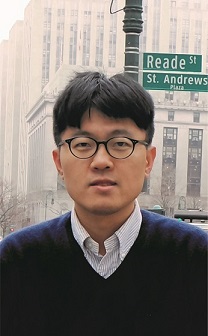
Dr. Changyeol Lee, Associate Professor at Department of Earth System Sciences, College of Science, Yonsei University
Northeast Asia may be one of the best natural laboratories to investigate both arc and intraplate volcanisms which have developed since the mid-Miocene. The arc volcanoes have occurred above the sinking young (Philippine) and old (Pacific) oceanic plates in Southwest and Northeast Japan subduction zones, respectively. The intraplate volcanoes across the Korean Peninsula and China have occurred above the stagnant Pacific plate in the mantle transition zone. What are the roles of the subducting oceanic plates beneath the arc and the stagnant slab in the mantle transition zone in generation of both types of volcanism? I will describe some of numerical modeling studies of our research group to explain them.
Earth is the only known planet where liquid water covers the vast area (e.g., ocean) and has been circulating from the surface to deep mantle for billions of years through subduction. Since the oceanic plate was formed at the mid-ocean ridge, its long-time submersion has hydrated the upper portion of the oceanic plate, expressed as hydrated rocks such as serpentinite. The hydrous rocks in the oceanic plate sink into the mantle at the trench and experience dehydration owing to increases in temperature and pressure, liberating aqueous fluids to the ambient oceanic crust and mantle. Existence of the aqueous fluids substantially decreases the rock solidi, causing slab melting (partial melting of the oceanic crust) and/or flux melting (partial melting of the mantle wedge). The magma formed by the melting processes ascends and finally erupts on the surface; arc volcanoes form in subduction zones.
Understanding arc volcanism in subduction zones has been earth scientists’ major research topic even before the modern plate tectonics was introduced. Among the subduction zones around the world, the Japan subduction zone is one of the most well-studied areas for decades. The subduction zone is decomposed into two end-member varieties of which current tectonic frameworks have been formed since the mid-Miocene (Kimura et al., 2005; Sdrolias and Müller, 2006); the Southwest and Northeast Japan subduction zones where the young Philippine oceanic plate (~15 Ma) and old Pacific oceanic plate (~130 Ma) sink at the Nankai trough and Japan trench, respectively. These subduction zones are excellent natural laboratories because the effects of the young and old slabs on the arc volcanism can be investigated and compared with each other. Along with the arc volcanism, the intraplate volcanism in Korea and Eastern China occurred during the Quaternary is thought to be related to the specific processes happened in the stagnant slab of the mantle transition zone (MTZ). My research group has especially focused on the roles of water in the arc volcanism in the Japan subduction zone and the intraplate volcanism in Korea; the details are summarized below.
1. Southwest Japan
The Southwest Japan subduction zone is well known for the Quaternary adakite volcanism resulted from slab melting (partial melting of the eclogited oceanic crust) (Kimura et al., 2005; Peacock and Wang, 1999). Since the subduction was initiated by the converging Philippine oceanic plate at ~17 Ma, the forearc-to-arc volcanic front migration has simultaneously occurred, coeval with the volcanism of the mid-Miocene high-magnesium andesite (HMA) (~15–12 Ma), scattered monogenic volcanism (~12–4 Ma), and Quaternary adakite volcanism (< ~2 Ma) (Kimura et al., 2005). The volcanic front migration implies the deepening mechanical decoupling owing to the downward growth of the weak hydrous layer (e.g., serpentinite) at the slab interface, leading to the conversion of the hot forearc mantle to cold mantle (i.e., transition from subduction infancy to mature subduction) (Agard et al., 2016).
Despite this important implication, however, no quantitative study has been conducted to explain the volcanic front migration in the Southwest Japan subduction zone. Thus, a series of 2-dimensional numerical models were conducted to quantitatively demonstrate the self-consistent formation of the weak hydrous layer with the permeability anisotropy (Lee and Kim, under review), advanced from my previous study (Lee and Kim, 2021). The model results show that the spontaneous downdip growth of the weak hydrous layer at the slab interface converts the hot forearc mantle to cold mantle, explaining the forearc-to-arc volcanic front migration and the coeval magmatism since the subduction initiation at ~17 Ma (Fig. 1). Our results confirm that the permeability anisotropy of the serpentinite (Kawano et al., 2011) in the weak hydrous layer is a key controlling factor which leads to an extension of the weak layer toward the tip of the mantle wedge. The results also explain the present-day geophysical and geochemical observations in the forearc, including the nonvolcanic seismic tremors (Obara, 2002), trench-parallel seismic anisotropy (Salah et al., 2008), and slab/mantle-derived geochemical components in deep groundwater (Morikawa et al., 2016).
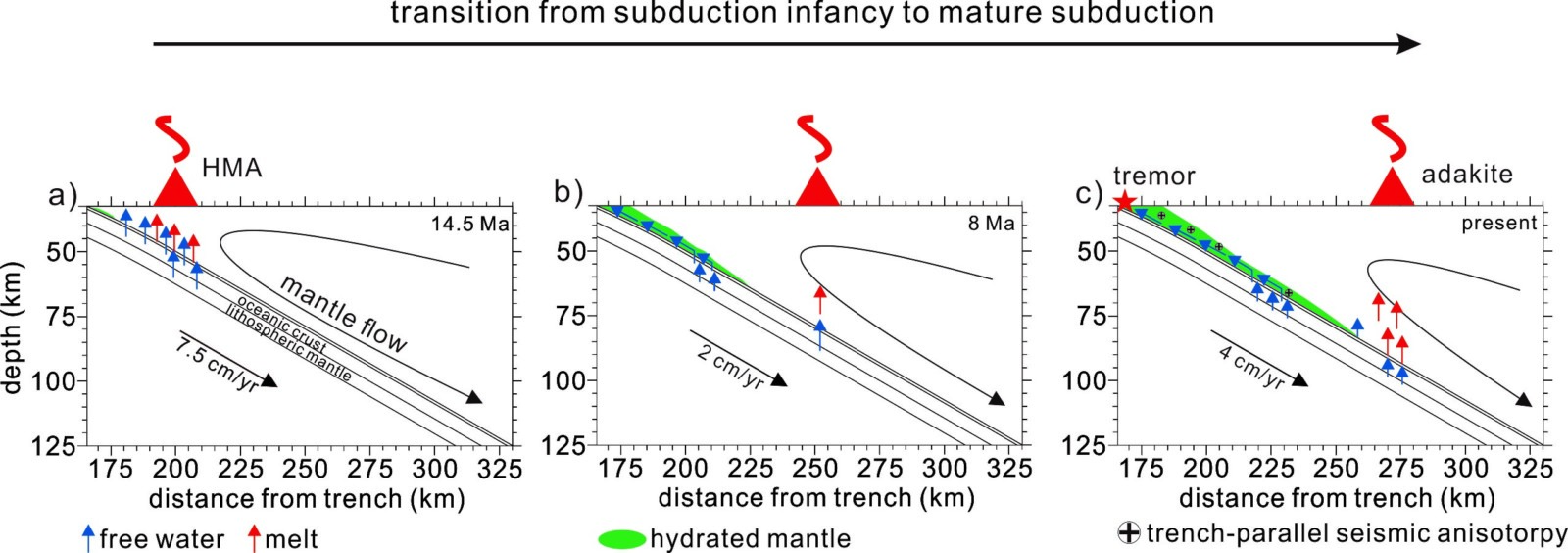
Figure 1. Schematic descriptions of the major geophysical and geochemical observations correlated with the formation of the weak hydrous layer at the slab interface in the Southwest Japan subduction zone. a) During the early stage of the subduction, slab dehydration mostly occurs at the sub-forearc slab and results in the slab melting, explaining the high-magnesium andesite (HMA) in the forearc. Blue and red arrows indicate the flow direction of the free water and melt, respectively. The green zone indicates the weak hydrous layer at the slab interface. b) Ongoing downward formation of the weak hydrous layer at the slab interface which decouples the mantle from the subducting slab toward the arc. The foliated serpentinite in the weak hydrous layer detours the free water expelled from the slab toward the tip of the mantle wedge. The less slab-derived free water owing to the slow subduction results in less flux melting, forming the scattered monogenetic forearc and arc magmatism. c) The present formation of the weak hydrous layer at the slab interface which detours the free water toward the tip of the mantle wedge, explaining the non-volcanic seismic tremor and trench-parallel seismic anisotropy as well as groundwater geochemistry in the forearc. The slab melting by the slab-derived free water from the underlying lithosphere results in the Quaternary adakite volcanism. (Modified from Lee and Kim, under review).
2. Northeast Japan
Compared to relatively rare and scattered arc volcanism in the Southwest Japan subduction zone, the Northeast Japan subduction zone has experienced extensive arc volcanism for the tens of million years (Yoshida et al., 2014). One interesting observation is that the clustered (grouped) arc volcanoes overly the ‘hot fingers’ in the mantle wedge, which are extended from the sub-arc to sub-backarc mantle (Tamura et al., 2002). A recent numerical study quantitatively shows how the hot fingers have formed in the mantle wedge since the cessation of the backarc opening of the East Sea (Sea of Japan) in the mid-Miocene (Lee and Wada, 2021).
The hot fingers in the mantle wedge fluxed by the liberated water from the underlying slab develop the sub-arc to sub-backarc melt distribution (Yoo and Lee, 2020), consistent with the high-resolution seismological studies on the mantle wedge (Hasegawa, 2018; Nakajima et al., 2013). However, most of the arc volcanoes are distributed along the arc except for rare backarc volcanoes. This indicates that melt in the sub-backarc mantle should be transported toward the sub-arc mantle; melt focusing beneath the arc volcanoes should exist.
In a recent study of my research group, the partial melting and melt transport in the mantle wedge beneath the Northeast Japan subduction zone were quantitatively investigated using a series of two-dimensional numerical models with controlling factors like melt viscosity, melt density, mechanical decoupling depth at the slab interface, and melt freezing (Yoo and Lee, under review). Compared with the previous studies which considered the behavior of the aqueous fluids only, we quantitatively calculated partial melting of the hydrated mantle wedge and melt transport controlled by melt buoyancy and corner flow of the mantle wedge. Model results show that the melt focusing in the Northeast Japan subduction zone is formed by the solid-state corner flow and melt freezing in the mantle wedge (Fig. 2), explaining the geographically focused arc volcanoes, seismic tomography, and geochemically estimated melt production (Kimura and Nakajima, 2014) in this subduction zone.
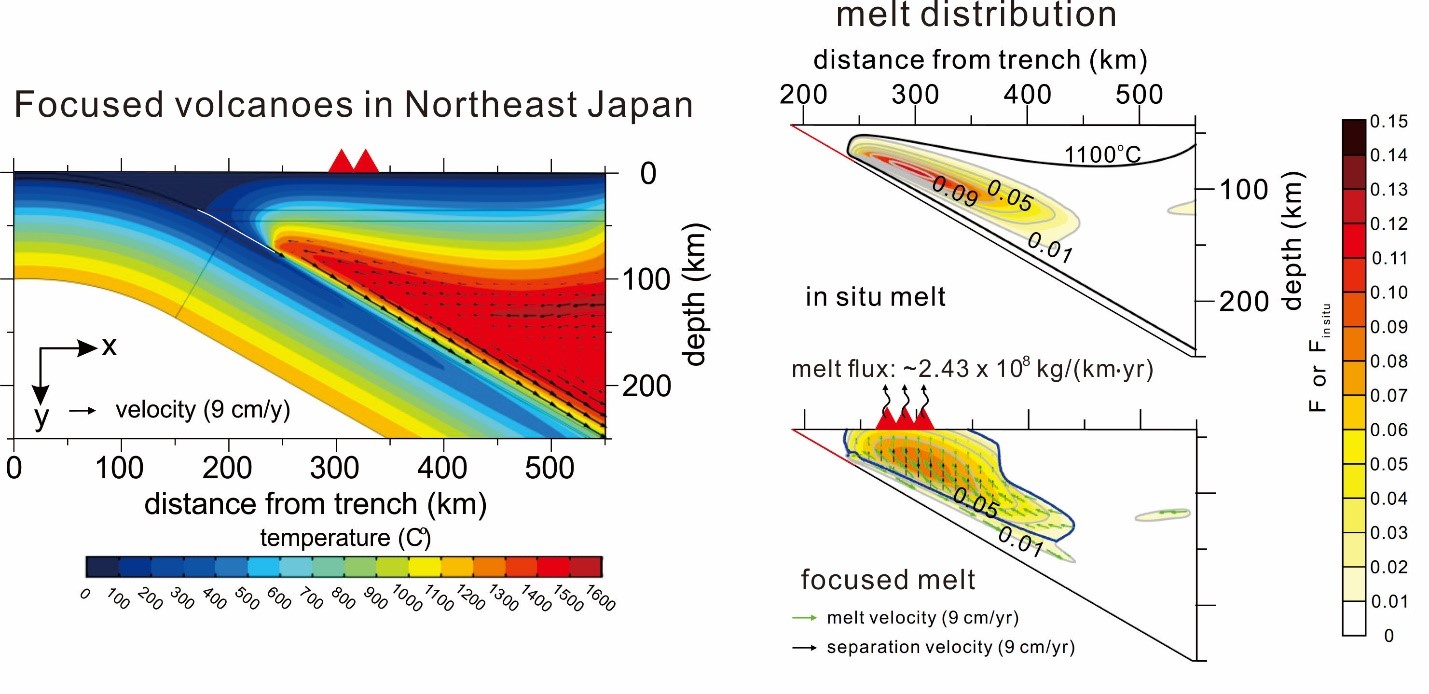
Figure 2. Distributions of temperature, velocity, and melt calculated from our numerical model study. Left: Semi-steady-state distribution of temperature and velocity beneath the focused arc volcanoes in Northeast Japan. Right: Distributions of in-situ and dynamically calculated melt. The melt dragged by the corner flow is focused to the sub-arc mantle. (Modified from Yoo and Lee, under review)
3. Korea
Quaternary intraplate volcanoes are scattered throughout Northeast Asia including Eastern China and Korean Peninsula. In some early studies, the volcanoes were thought to result from ordinary ‘hot’ mantle plumes (e.g., thermal plumes rooted in the lower mantle) (e.g., Turcotte and Schubert, 2002). However, high-resolution seismic tomographic studies (Zhao and Tian, 2013; Zhao et al., 2009) have shown that the low-velocity zone is confined to the mantle over the subducted Pacific plate in the mantle transition zone (i.e., stagnant slab); no rooted mantle plume exists in the lower mantle. The volcanic rock geochemistry indicates the ordinary mantle temperature (~1400 °C) for the partial melting (Kuritani et al., 2019); hot plumes are unlikely. Thus, alternative ideas have been suggested for the intraplate volcanism and here I discuss the wet plume hypothesis by focusing on the fate of the hydrous minerals in the stagnant slab.
Previous studies show that the upper layer of the old Pacific plate can hold relatively large amount of hydrous minerals, which were transported to the mantle transition zone (Lee and Kim, 2021; van Keken et al., 2011). The hydrated layer of the stagnant slab was detached and ascended upward owing to its low density and viscosity (i.e., Rayleigh-Taylor instability), forming wet (hydrous) plumes of which temperature is even lower than the ambient mantle (Richard and Bercovici, 2009; Richard and Iwamori, 2010). The ascending wet plumes reach the lithosphere base and form melts for the intraplate volcanism. Although the studies suggested a potential explanation for the intraplate volcanoes, the spacing and timing of the wet plumes cannot constrain the sparsely distributed volcanoes (distances: ~330–563 km) and recent eruption timing (<~2 Ma) in Korea.
To explain the intraplate volcanism in Korea, a series of two-dimensional numerical models (Kim et al., 2022) were constructed by considering the partial hydration of the mantle transition zone and the olivine-wadsleyite phase transition at the 410-km discontinuity, both of which have not been considered in the previous studies of wet plumes. The results showed that the wet plumes originating from the hydrated layer of the stagnant slab were retarded at the phase transition zone (Fig. 3). After several millions of years’ retardation, the wet plumes enter the overlying asthenospheric mantle, forming mixed components of the hydrous layer and mantle transition zone, named as the hybrid plumes. The observed spacing (~485 km) and elapsed time (~24 Myr) of the hybrid plumes to reach the lithosphere base explain the spatiotemporal observations of the intraplate volcanoes. Furthermore, the mixed components of the hybrid plumes explain the spatial distribution of water in the mantle transition zone beneath Korea (Kelbert et al., 2009). This study shows important roles of the hydrated mantle transition zone and phase transition in the intraplate volcanism in Northeast Asia.
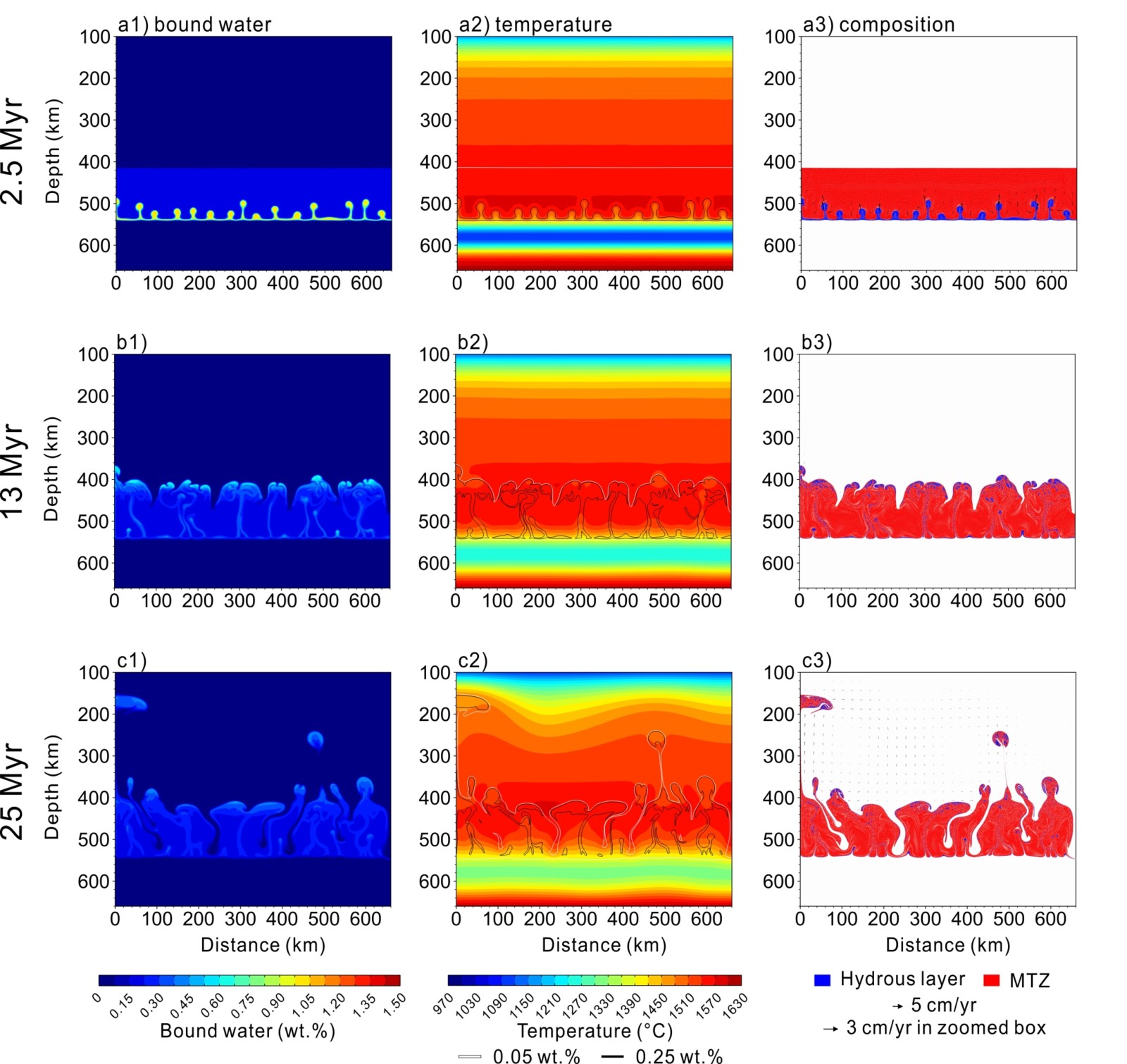
Figure 3. Distributions of the bound water, temperature, and hydrous minerals for the reference experiment. a–c) Results from the reference experiment at 2.5, 13.0, and 25.0 Myr, respectively. Left column: Distribution of the bound water (wt.%). Middle column: Temperature (°C) in the model domain. The black and white contours correspond to 0.25 and 0.05 wt.% bound water, respectively, for a clear view of wet and hybrid plumes in the mantle transition zone and asthenospheric mantle, respectively. Right column: Distribution of the hydrous layer of the stagnant slab (blue particle tracers) and hydrous mantle in the mantle transition zone (red particles). (Modified from Kim et al., 2022)
4. Prospect
Although the studies described above provide us important insights into the arc and intraplate volcanism in Northeast Asia, there are still unconsidered processes, which could substantially affect the model calculations. One is the compaction pressure which results in time-evolving transport of the aqueous fluids and melts in the mantle (e.g., Cerpa et al., 2017; Wilson et al., 2014). The role of the compaction pressure in the behaviors of the aqueous fluids and melts should be tackled through numerical modeling. The other is a large difference of the water solubilities between the mantle transition zone and overlying asthenospheric mantle bounded by the 410-km discontinuity. Previous studies show that the water solubility of the mantle transition zone ranges from ~1 to 3 wt% (e.g., Kohlstedt et al., 1996) which is an order larger than that of the asthenospheric mantle (~0.15 wt%) (Hirschmann et al., 2005). The significant reduction of the water solubility across the 410-km discontinuity releases aqueous fluids, which results in the melt layer on the discontinuity (Bercovici and Karato, 2003). This indicates that the wet plume which has ~1 wt% water should experience substantial dehydration when it passes through the 410-km discontinuity, releasing aqueous fluids to the ambient mantle. Thus, a kind of ‘flux’ melting at this depth is expected to occur, and the fate of the melt should be controlled by the melt parameters such as density and viscosity as well as the compaction pressure. We are currently working on the roles of the compaction pressure and decreases in water solubility in the melt formation and behavior to further improve our understanding of the arc and intraplate volcanism in Northeast Asia.
References Agard, P., Yamato, P., Soret, M., Prigent, C., Guillot, S., Plunder, A., Dubacq, B., Chauvet, A., Monié, P., 2016. Plate interface rheological switches during subduction infancy: Control on slab penetration and metamorphic sole formation. Earth and Planetary Science Letters 451, 208-220.Bercovici, D., Karato, S.-i., 2003. Whole-mantle convection and the transition-zone water filter. Nature 425, 39-44. Cerpa, N.G., Wada, I., Wilson, C.R., 2017. Fluid migration in the mantle wedge: Influence of mineral grain size and mantle compaction. Journal of Geophysical Research: Solid Earth 122, 6247-6268. Hasegawa, A., 2018. Seismic imaging of mantle wedge corner flow and arc magmatism. Proceedings of the Japan Academy, Series B 94, 217-234. Hirschmann, M.M., Aubaud, C., Withers, A.C., 2005. Storage capacity of H2O in nominally anhydrous minerals in the upper mantle. Earth and Planetary Science Letters 236, 167-181. Kawano, S., Katayama, I., Okazaki, K., 2011. Permeability anisotropy of serpentinite and fluid pathways in a subduction zone. Geology 39, 939-942. Kelbert, A., Schultz, A., Egbert, G., 2009. Global electromagnetic induction constraints on transition-zone water content variations. Nature 460, 1003-1006. Kim, H., Lee, Y., Kim, D., Lee, C., 2022. Roles of partial hydration of mantle transition zone and wadsleyite–olivine phase transition in wet plume development: Origin of Quaternary intraplate volcanoes in Korea. Tectonophysics 837, 229467. Kim, Y., Lee, C., Kim, S.-S., 2015. Tectonics and volcanism in East Asia: Insights from geophysical observations. Journal of Asian Earth Sciences 113, 842-856. Kimura, J.-I., Nakajima, J., 2014. Behaviour of subducted water and its role in magma genesis in the NE Japan arc: A combined geophysical and geochemical approach. Geochimica et Cosmochimica Acta 143, 165-188. Kimura, J.-I., Stern, R.J., Yoshida, T., 2005. Reinitiation of subduction and magmatic responses in SW Japan during Neogene time. Geological Society of America Bulletin 117, 969-986. Kohlstedt, D.L., Keppler, H., Rubie, D.C., 1996. Solubility of water in the α, β and γ phases of (Mg,Fe)2SiO4. Contributions to Mineralogy and Petrology 123, 345-357. Kuritani, T., Xia, Q.-K., Kimura, J.-I., Liu, J., Shimizu, K., Ushikubo, T., Zhao, D., Nakagawa, M., Yoshimura, S., 2019. Buoyant hydrous mantle plume from the mantle transition zone. Scientific Reports 9, 6549. Lee, C., Kim, Y., 2021. Role of warm subduction in the seismological properties of the forearc mantle: An example from southwest Japan. Sci Adv 7, eabf8934. Lee, C., Wada, I., 2021. Volcano Clustering Promoted by the Cessation of Back-Arc Spreading and Ensuing Nascent Lithospheric Drips. Geophysical Research Letters n/a, e2020GL091433. Morikawa, N., Kazahaya, K., Takahashi, M., Inamura, A., Takahashi, H.A., Yasuhara, M., Ohwada, M., Sato, T., Nakama, A., Handa, H., Sumino, H., Nagao, K., 2016. Widespread distribution of ascending fluids transporting mantle helium in the fore-arc region and their upwelling processes: Noble gas and major element composition of deep groundwater in the Kii Peninsula, southwest Japan. Geochimica et Cosmochimica Acta 182, 173-196. Nakajima, J., Hada, S., Hayami, E., Uchida, N., Hasegawa, A., Yoshioka, S., Matsuzawa, T., Umino, N., 2013. Seismic attenuation beneath northeastern Japan: Constraints on mantle dynamics and arc magmatism. Journal of Geophysical Research: Solid Earth 118, 5838-5855. Obara, K., 2002. Nonvolcanic Deep Tremor Associated with Subduction in Southwest Japan. Science 296, 1679-1681. Peacock, S.M., Wang, K., 1999. Seismic consequences of warm versus cool subduction metamorphism: Examples from southwest and northeast Japan. Science 286, 937-939. Richard, G.C., Bercovici, D., 2009. Water-induced convection in the Earth's mantle transition zone. Journal of Geophysical Research: Solid Earth 114, B01205. Richard, G.C., Iwamori, H., 2010. Stagnant slab, wet plumes and Cenozoic volcanism in East Asia. Physics of the Earth and Planetary Interiors 183, 280-287. Salah, M.K., Seno, T., Iidaka, T., 2008. Upper mantle anisotropy beneath central and southwest Japan: An insight into subduction-induced mantle flow. Journal of Geodynamics 46, 21-37. Sdrolias, M., Müller, R.D., 2006. Controls on back-arc basin formation. Geochemistry Geophysics Geosystems 7. Tamura, Y., Tatsumi, Y., Zhao, D., Kido, Y., Shukuno, H., 2002. Hot fingers in the mantle wedge: new insights into magma genesis in subduction zones. Earth and Planetary Science Letters 197, 105-116. Turcotte, D., Schubert, G., 2002. Geodynamics. Cambridge Univ. Press, Cambridge, 2nd Ed. van Keken, P.E., Hacker, B.R., Syracuse, E.M., Abers, G.A., 2011. Subduction factory: 4. Depth-dependent flux of H2O from subducting slabs worldwide. Journal of Geophysical Research: Solid Earth 116. Wilson, C.R., Spiegelman, M., van Keken, P.E., Hacker, B.R., 2014. Fluid flow in subduction zones: The role of solid rheology and compaction pressure. Earth and Planetary Science Letters 401, 261-274. Yoo, S., Lee, C., 2020. Correlation of Quaternary Volcano Clusters With Partial Melting of Mantle Wedge, Northeast Japan: A Numerical Model Study. Geophysical Research Letters 47. Yoshida, T., Kimura, J.-I., Yamada, R., Acocella, V., Sato, H., Zhao, D., Nakajima, J., Hasegawa, A., Okada, T., Honda, S., Ishikawa, M., Prima, O.D.A., Kudo, T., Shibazaki, B., Tanaka, A., Imaizumi, T., 2014. Evolution of late Cenozoic magmatism and the crust–mantle structure in the NE Japan Arc. Geological Society, London, Special Publications 385, 335-387. Zhao, D., Tian, Y., 2013. Changbai intraplate volcanism and deep earthquakes in East Asia: a possible link? Geophysical Journal International. Zhao, D., Tian, Y., Lei, J., Liu, L., Zheng, S., 2009. Seismic image and origin of the Changbai intraplate volcano in East Asia: Role of big mantle wedge above the stagnant Pacific slab. Physics of the Earth and Planetary Interiors 173, 197-206.