Have you ever wondered how Earth became habitable. In this week’s news and views, we have Fabio Capitanio, Associate Professor at Monash University sharing insights into the role Geodynamics in the evolution of Earth and life on it.
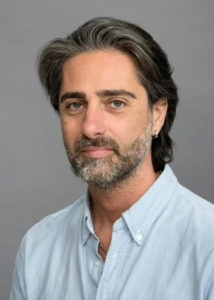
Dr. Fabio Capitanio, Associate Professor and ARC Future Fellow at School of Earth, Atmosphere and Environment, Faculty of Science, Monash University.
Our planet has unique features which make it suitable for life. Understanding how the Earth became habitable is necessary to answer the most fundamental questions on the evolution of Earth and life on it. But not only. The ability to interpret our planet’s evolution may unlock the key to the habitability of other rocky exoplanets out there, where similar conditions, or lack thereof, may have occurred in the geological past. Understanding the evolutionary path of planets drives our strategies for exploration of the outer space, in the attempt to answer the ultimate question: where do we come from?
Life on Earth is the result of unique conditions that led to the formation of an atmosphere. The evolution of the atmosphere is coupled to that of the mantle and the continents, through progressive magmatism, differentiating mantle and crust, and exchanging gasses within the interior and exterior of the solid Earth. Fundamental atmospheric compounds in various forms, most importantly N2 and O2, others necessary to life, such as C, or relevant to temperature control, that is, the range of greenhouse gases, from CO2 to CH4, are all regulated through tectonics: major outgassing, or atmospheric input, occurs at rift zones, mid-ocean ridges, at site of large igneous province emplacement and at volcanic arcs, along convergence zones; these may cycle through exogenic reservoirs, the hydro-, bio- and atmosphere, to be ultimately sequestered and returned to the planetary interior by subduction of the lithosphere, accumulated in the crust, serpentinized mantle, and deep-sea sediments.
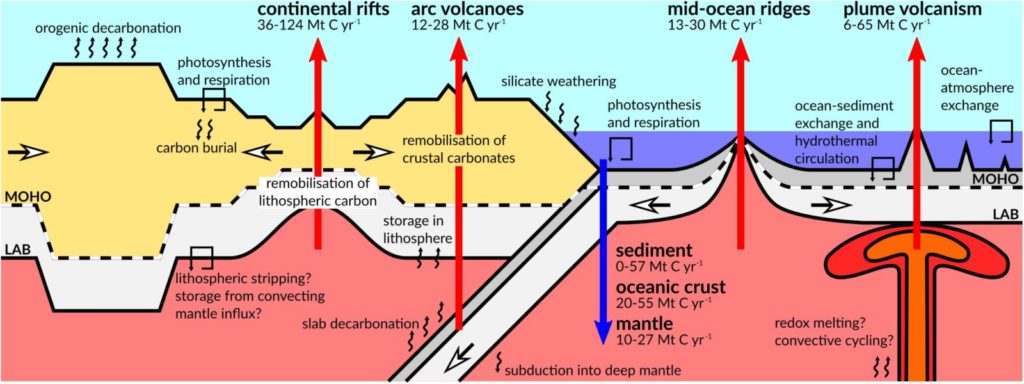
Cartoon illustrating major fluxes of carbon to, from, and within the Earth’s exogenic and endogenic systems. Source: Wong et. al., 2018
It is well known that the environments where crust and mantle are formed are set in their tectonic context, describing how these processes link across scales, from the pressure-temperature conditions for magmatic evolution, to the lithospheric structures providing the boundary conditions, to the large-scale frame of tectonic features, referred to as the global tectonics. This strongly suggests that the atmosphere-and-life and tectonics-and-planetary geodynamics systems must be coupled, with conditions for the evolution of life and its diversification dictated by the geodynamics of a convecting planet. Consequently, several attempts can be made to depict an evolutionary sketch for our planet, from infancy to habitability, through the evolution of their global tectonic regimes.
The most illustrative point to start from is the present-day, drawing our inferences backwards, á la “uniformitarian” way. In the present day, our planet is in the plate tectonics regime. This assumes that plates, or, better, the oceanic lithospheres, are relatively rigid and move coherently from mid-ocean ridges, where they form, to convergent margins, where they are recycled in the mantle. Then, what would be the net flux of gasses is a matter of balancing mid-ocean ridges and convergent margins lengths.
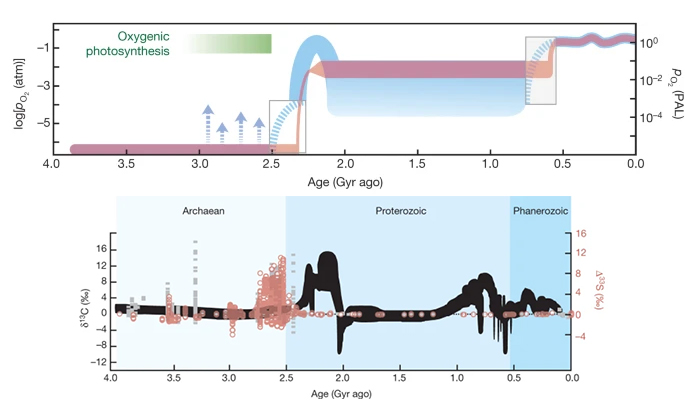
[Top] Evolution of Earth’s atmospheric oxygen content through time. [Bottom] Summary of Carbon and Sulphur isotope data through Earth’s history. Source: Lyon et al., 2014
This approach has been proven viable and estimates of net rates of CO2 since the Mesozoic era [1] can be reasonably constrained by plate kinematics reconstructions, where the varying length of the ridges and subduction zones in time are reconstructed. This approach reveals the long- and short-term impact of plate margins formation and migration on CO2 flux and, thereby, greenhouse conditions, positively correlating with major cooling/warming event in the Mesozoic. CO2 is central to understand average atmospheric temperatures and inferences to paleoclimate’s thermal optima and maxima, however, when it comes to deep time, that is before ~540 Ma, the interest shifts to free oxygen, as a proxy for the raise of the atmosphere itself. Similar tools can be devised, addressing the long-term evolution of atmospheric oxygen content w.r.t today, using the same concepts borrowed form geodynamics. Rigid plates somehow imply a rather neutral net O2 flux over long timescales, enforcing the equivalence between spreading and subduction rates. In absence of plate kinematic constraints for the Precambrian, this reasonable assumption explains the rather constant value of atmospheric pO2 between the Global Oxidation Event, (GOE), ~2.5 Ga, and the Neoproterozoic Oxygenation Event (NOE), 800-540 Ma [2] (Figure 2).
What is the cause of the step-like increase of atmosphere oxygenation remains less constrained, and rather enigmatic. The most recent oxygen content jump, associated with the NOE, may have been caused by the remobilization of the continental carbon reservoir through metamorphic and magmatic decarbonation of the crust [3], then followed by relatively smaller increments due to biogenic cycles to reach current values [4].However, how to interpret the evolution prior to this time and the rapid transition from negligible oxygen content to a 10 to 104-fold increase across the GOE, remains speculative, mostly for the uncertainties in the tectonics mode of the early Earth. This is still a very hotly debated topic as how tectonics worked in the early stages of the planet’s evolution remains difficult to constrain.
Traces of the original tectonics are now found disseminated in some remnants of the early continents, the cratons, where a diverse, although cryptical, record of coupled tectonic evolution of the surface, differentiation of the mantle, formation of the atmosphere and evolution of life, through time, is kept.
The early continents differentiated progressively from the mantle through extraction of juvenile magmatic crust, increasing their buoyancy while differentiating, originating the first topography [5]. Recycling and reworking of crust and mantle are common features of the first continents, likely more vigorous in the early stages of the cratons, as suggested by the zircon record, and variously disseminated throughout the cratogenesis, until becoming inefficient to the point to allow lithospheric keels to strengthen and stabilize. The environments in which crust and mantle formed and evolved were not different from present-day’s and are akin to rifts and subduction zones. However, unlike modern plate tectonics, these margin-like environments did not last long, and did not allow fully-fledged plate tectonics to develop, due to the inherent stiffening of large portions of the mantle as thick magmatic crust is extracted [6]. These environments are critical to the Earth’s habitability and their role is revealed by the way surface tectonics links to the mantle dynamics and, therefore, to the exchange between endo- and exogenic volatile reservoirs.
The subduction of cold lithosphere, the fundamental tenet of plate tectonics, must be ruled out in the Archean, by several arguments. Subduction is a sink for atmospheric oxygen, therefore a steady and ongoing subduction prior to the GOE must imply larger initial O2 level, that is, the level did not change in time. This is indisagreement with the observation: the redox state of Archean komatiites [7] indicates a state very close to present-day basalts, and a mantle oxygen decrease to current content of –0.02 ‰ [8], minor respect to the mantle mass, yet sufficient to replenish a relatively thin atmosphere layer. Subduction is also a mantle stirring mechanism, so that the relatively poor mixing of the Archean mantle [9] suggests that the surface features, albeit similar to those along present-day plate margins, could not be part of convection, through deep lithosphere subduction. Because it is the engine of plate tectonics, without subduction going, a global plate tectonics network would have been difficult to propel in the Archean.
So, what was the tectonic regime of the early Earth and how it helped habitability? From the simple atmospheric oxygen content argument, the GOE major outgassing requires that the source outpaced the sink, which may be achieved if a global set of rifts-plumes dominated tectonics, contributing to planet outgassing, whereas there is no need for mechanisms for sequestration of large gas volumes, i.e., subduction. This depicts an image of an early Earth that has some form of vigorous tectonics, not a stagnant lid, nor plate tectonics, likely sluggish or squishy, dominated by magmatism and mantle thermochemical differentiation [10]. Tectonic features of the early Earth are those of related to plume impingement, or deep lithospheric removal [11], although the volumetric contribution to cratons thickening remains difficult to constrain, or large-scale rifting, which reproduces Hadean crustal volume formation trends [12] and allows the formation of large volumes of depleted, buoyant keels [13] comparable to the preserved cratons. Yet, the question remains, how “global” was this collection of environments? Are the craton remnants – and most importantly their tectonics – representative of the whole Earth? The oxygen content record does not show any sign of major increase in the early stage, however from 3 Ga several oxygen “whiffs” are recorded in the Western Australian’s shales preceding the main atmosphere forming GOE, with episodic increase up to a 10 -103-fold increase. Although these are relatively less constrained in duration and magnitude, the whiffs are synchronous to a sediment sulphur content spike, then disappearing during the GOE, supporting subaereal conditions for oxidation – of pyrite and other sulphides –and riverine transport systems, then followed by an increase of carbon in the sedimentary record, indicative of sequestration (Figure 2), that is, topography. Such early environments may be harbinger of the beginning of a tectonics revolution and large oxygenation of the atmosphere, starting with a rift.
Other alternatives may exist. These include geochemical processes, such as the enhanced early oxygen sequestration by a predominantly mafic crust prior to 3 billion years ago [14], or emphasise the role of oxygenic photosynthesis, although without accumulation in the atmosphere [15], or may be as provocative as explaining step-like growth of atmospheric oxygen entirely through the biogeochemical cycles of carbon, oxygen and phosphorus [16]. In fact, the scarcity of data on the early Earth’s atmosphere and life does not safely allows considering our views conventional and many will be challenged. However, others will not: The planetary interiors’ geodynamics ultimately propelled the evolution of the third stone from the Sun into the blue planet.
References
- Müller, R.D., B. Mather, and A.e.a. Dutkiewicz, Evolution of Earth’s tectonic carbon conveyor belt. Nature, 2022. 605: p. 629–639.
- Lyons, T., C. Reinhard, and N. Planavsky, The rise of oxygen in Earth’s early ocean and atmosphere. Nature, 2014. 506: p. 307–315.
- Lee, C.-T.A., et al., Two-step rise of atmospheric oxygen linked to the growth of continents. Nature Geosci., 2016. 9: p. 417-423.
- Catling, D.C. and K.J. Zahnle, The Archean atmosphere. Sci. Adv., 2020. 6.
- Chowdhury, P., et al., Magmatic thickening of crust in non–plate tectonic settings initiated the subaerial rise of Earth’s first continents 3.3 to 3.2 billion years ago. Proc. Nat. Am. Soc., 2021. 118.
- Capitanio, F.A., N. O., and P. Cawood, Thermochemical lithosphere differentiation and the origin of cratonic mantle. Nature, 2020. 588: p. 89-94.
- Canil, D., Vanadium partitioning and the oxidation state of Archaean komatiite magmas. Nature, 1997. 389: p. 842–845.
- Bindeman, I.N., et al., Oxygen isotope (δ18O, Δ′17O) insights into continental mantle evolution since the Archean.Nat. Commun., 2022. 13(3779).
- Debaille, V., et al., Stagnant-lid tectonics in early Earth revealed by 142Nd variations in late Archean rocks.Earth Plan. Sci. Let., 2013. 373: p. 83-92.
10. Lenardic, A., The diversity of tectonic modes and thoughts about transitions between them. Phil. Trans. R. Soc. A, 2018. 376: p. 20170416.
11. Gerya, T.V., Precambrian geodynamics: Concepts and models. Gond. Res., 2014. 25(2): p. 442-463.
12. Capitanio, F.A., et al., Reconciling thermal regimes and tectonics of the early Earth. Geology, 2019. 47: p. https://doi.org/10.1130/G46239.1.
13. Capitanio, F.A., et al., Craton formation in early Earth mantle convection regimes. J. Geophys. Res., 2022. 127(4).
14. Smit, M. and K. Mezger, Earth’s early O2 cycle suppressed by primitive continents. Nature Geosci., 2017. 10: p. 788–792.
15. Crowe, S. and e. al., Atmospheric oxygenation three billion years ago. Nature, 2013. 501: p. 535–538.
16. Alcott, L.J., B.J. Mills, and S.W. Poulton, Stepwise Earth oxygenation is an inherent property of global biogeochemical cycling. Science, 2019. 366(6471): p. 1333-1337.