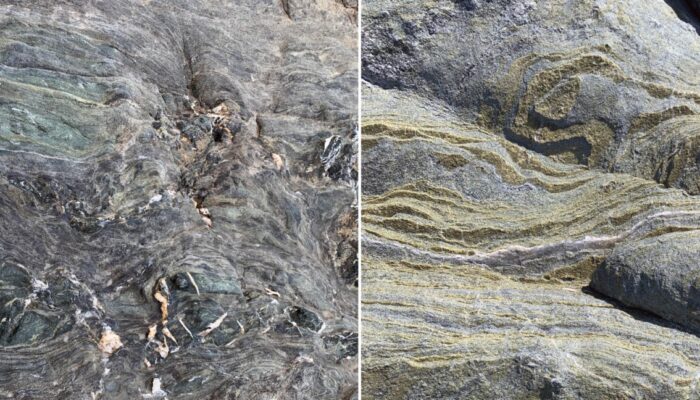
Subduction zones are dynamic regions where intense geological processes like earthquakes, volcanic eruptions, and the recycling of oceanic crust are constantly at play. A key factor that influences the behavior of these zones is *interface rheology*—the strength and viscosity of the boundary (the interface) between the subducting and overriding plates. Understanding this interface is crucial for interpreting both the short- and long-term dynamics of subduction systems. In this week’s blog post, Ana Lorena Abila, a PhD student at ETH Zürich, will introduce her research on modelling subduction shear zones.
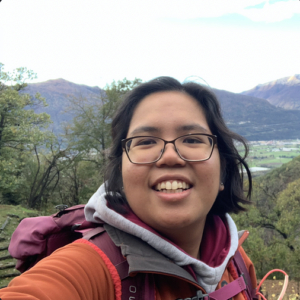
Ana Lorena Abila is a 3rd year PhD student in the Structural Geology and Tectonics Group at ETH Zürich. Her research is focused on quantifying the strength of the deep subduction interface by combining numerical models, field observations, and deformation experiments.
How Do We Study Deep Subduction Interface Rheology?
Estimating the strength and viscosity of subduction interfaces is challenging, largely due to the wide variation in lithologies, interface thickness, pressure, and temperature conditions reported in both ancient and present-day subduction zones. Traditional methods approach this issue in several ways: (1) by measuring bulk interface strength from geophysical estimates like force-balance calculations and heat flow constraints, (2) by studying deformed rocks and using methods such as recrystallized grain size paleopiezometry, (3) by applying experimental flow laws for reported lithologies such as eclogite, and (4) by using large-scale geodynamic modeling to replicate observed behaviors. However, these approaches often simplify subduction interface strength and tend to overlook the geological complexity at play.
In our recent study published in Geology, we aimed to improve these estimates by examining exhumed deep subduction mélange shear zones, which preserve the rheological conditions “frozen” in these ancient subduction zones. First, we compiled a comprehensive database of rock types and block-and-matrix distributions from 17 different subduction shear zones worldwide to capture the range of materials likely present along subduction interfaces. Then, we applied numerical shear experiments to each shear zone model to calculate their bulk shear strengths and viscosities. By simulating deformation across these varied compositions, we quantify the range of viscosity in natural subduction shear zones, providing a more detailed look at the forces acting along the subduction interface.
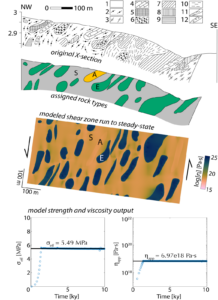
An example of the model setup based on a geological cross-section of the Makbal Eclogite unit, initially mapped by Sobolev et al. (1986) and Tagiri et al. (2010) and re-interpreted using reported lithologies and viscous rheologies. The original section was expanded into a rectangular model input, preserving the geometry, spatial relationships, and block distribution. The model was run for approximately 10ky, with steady-state deformation (black line) representing the rheological behavior of the shear zone. Key lithologies: 1 = alluvium, 2 = quartz-muscovite schist, 3 = garnet-chlorite schist, 4 = marble, 5 = fine-grained eclogite, 6 = garnet amphibolite, 7 = amphibolite (relict eclogite), 8 = amphibolite, 9 = garnet-albite-chlorite rocks, 10 = albite-chlorite rocks, 11 = talc rocks, 12 = carbonate-epidote-chlorite rocks. Implemented rheologies: A = aragonite, E = eclogite, S = wet quartz. Figure 1 from Abila et al. 2024.
How Strong Is the Deep Subduction Interface?
Our results reveal that the viscosity of natural subduction shear zones spans approximately 10^18 to 10^20 Pa⋅s, with shear strengths ranging from about 1 to 100 MPa. As would be expected, temperature emerged as a key factor in controlling viscosity across these zones, with higher temperatures generally correlating with lower viscosities. However, interestingly, even among shear zones deformed at similar temperatures, we observed up to a 50-fold variation in viscosity.
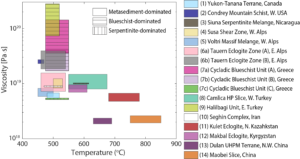
These are the results for the viscosities of the 17 modelled shear zones. Figure 2 from Abila et al. 2024.
This variability is largely due to differences in matrix composition, the width of shear zones, and the spatial distribution of blocks within each zone. Our findings capture the geological complexity of these shear zones and show how these variations critically influence the overall strength of the subduction interface.
What Do These Numbers Tell Us?
Our findings have significant implications for understanding subduction dynamics. While they confirm previous observations of a weak interface, our results also align with interface strength estimates from independent methods, like paleopiezometry and force-balance models. However, we observed that subduction interfaces cannot be considered uniformly weak, either downdip or across strike—a common assumption in larger-scale models. This confirms that heterogeneities in subduction inputs, such as sediment distributions and the presence or absence of basalt-dominated terranes (e.g. ridges or seamounts), can affect interface strength. Such variability might influence different scales of the subduction system, from large-scale plate velocity vectors, to earthquake cycles and variations in seismic energy release.
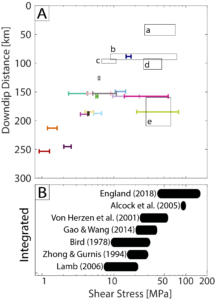
Here we compare our results with the (A) downdip (from paleopiezometry studies, shown as boxes) and (B) integrated shear stress estimates. Sources: (a) Koyama et al., 2024; (b) Behr & Platt, 2013; (c) Xia & Platt, 2017; (d) Schmidt & Platt, 2022; (e) Behrmann & Seckel, 2007. Figure 4 from Abila et al. 2024.
References
Abila, A. L., Behr, W. M., Ruh J. (2024). Strength of viscous subduction interfaces: A global compilation. Geology, https://doi.org/10.1130/G52518.1
Alcock, J., Arenas, R., & Martínez Catalán, J. R. (2005). Shear stress in subducting continental margin from high-pressure, moderate-temperature metamorphism in the Ordenes Complex, Galicia, NW Spain. Tectonophysics, 397(3–4), 181–194.
Behr, W. M., & Platt, J. P. (2013). Rheological evolution of a Mediterranean subduction complex. Journal of Structural Geology, 54, 136–155. https://doi.org/10.1016/j.jsg.2013.07.012
Behrmann, J. H., & Seckel, C. (2007). Structures, flow stresses, and estimated strain rates in metamorphic rocks of the Small Cyclades Islands Iraklia and Schinoussa (Aegean Sea, Greece). Geotectonic Research, 95(1), 1–11. https://doi.org/10.1127/1864-5658/07/0095-0001
Bird, P. (1978). Stress and temperature in subduction shear zones: Tonga and Mariana. Geophysical Journal International, 55(2), 411–434. https://doi.org/10.1111/j.1365-246X.1978.tb04280.x
England, P. (2018). On Shear Stresses, Temperatures, and the Maximum Magnitudes of Earthquakes at Convergent Plate Boundaries. Journal of Geophysical Research: Solid Earth, 123(8), 7165–7202. https://doi.org/10.1029/2018JB015907
Gao, X., & Wang, K. (2014). Strength of stick-slip and creeping subduction megathrusts from heat flow observations. Science, 345(6200), 1038–1041. https://doi.org/10.1126/science.1255487
Koyama, Y., Wallis, S. R., & Nagaya, T. (2024). Subduction plate interface shear stress associated with rapid subduction at deep slow earthquake depths: Example from the Sanbagawa belt, southwestern Japan. Solid Earth, 15(2), 143–166. https://doi.org/10.5194/se-15-143-2024
Lamb, S. (2006). Shear stresses on megathrusts: Implications for mountain building behind subduction zones. Journal of Geophysical Research, 111(B7), B07401. https://doi.org/10.1029/2005JB003916
Schmidt, W. L., & Platt, J. P. (2022). Stress, microstructure, and deformation mechanisms during subduction underplating at the depth of tremor and slow slip, Franciscan Complex, northern California. Journal of Structural Geology, 154, 104469. https://doi.org/10.1016/j.jsg.2021.104469
Sobolev, N. V., Dobretsov, N. L., Bakirov, A. B., & Shatsky, V. S. (1986). Eclogites from various types of metamorphic complexes in the USSR and the problems of their origin. In Geological Society of America Memoirs (Vol. 164, pp. 349–364). Geological Society of America. https://doi.org/10.1130/MEM164-p349
Tagiri, M., Takiguchi, S., Ishida, C., Noguchi, T., Kimura, M., Bakirov, A., Sakiev, K., Takahashi, M., Takasu, A., Bakirov, A., Togonbaeva, A., & Suzuki, A. (2010). Intrusion of UHP metamorphic rocks into the upper crust of Kyrgyzian Tien-Shan: P-T path and metamorphic age of the Makbal Complex. Journal of Mineralogical and Petrological Sciences, 105(5), 233–250. https://doi.org/10.2465/jmps.071025
Von Herzen, R., Ruppel, C., Molnar, P., Nettles, M., Nagihara, S., & Ekström, G. (2001). A constraint on the shear stress at the Pacific‐Australian plate boundary from heat flow and seismicity at the Kermadec forearc. Journal of Geophysical Research: Solid Earth, 106(B4), 6817–6833. https://doi.org/10.1029/2000JB900469
Xia, H., & Platt, J. P. (2017). Structural and rheological evolution of the Laramide subduction channel in southern California. Solid Earth, 8(2), 379–403. https://doi.org/10.5194/se-8-379-2017
Zhong, S., & Gurnis, M. (1994). Controls on trench topography from dynamic models of subducted slabs. Journal of Geophysical Research: Solid Earth, 99(B8), 15683–15695. https://doi.org/10.1029/94JB00809