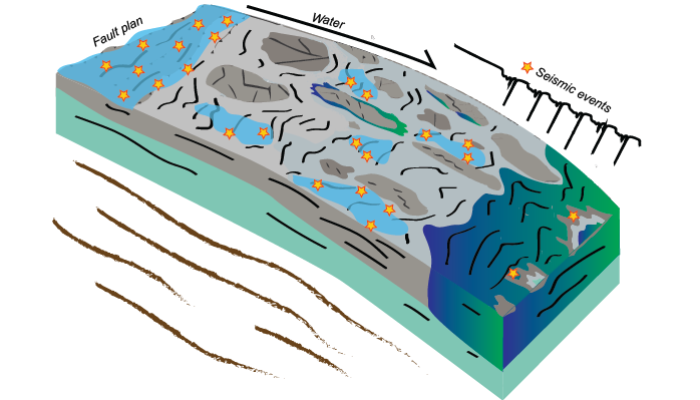
What is the role of fluids in seismic cycles ?
In this new blog post, I am really excited to share with you some of the results of my PhD, showing how we can use a continuum-based code with solid-fluid coupling to model seismic cycles. Our approach can lead (and hopefully it will lead) to better understand the effects of fluids on the long-term geodynamic processes. The code we developed can also allow us to explore seismicity and geodynamic processes within the same framework. Sounds exciting, right? I think so too!
Why are fluids important for seismicity?
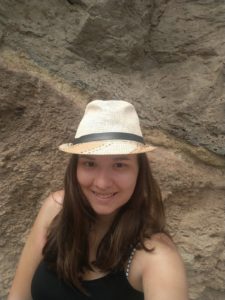
Betti Hegyi is currently in her second year of doctoral studies at ETH Zürich, in the Structural Geology and Tectonics (SGT) group. The work of modeling fluid-driven seismicity leads her to use a multidisciplinary approach and to establish new collaborations between different groups (e.g. SGT, Seismology and Geodynamics Group, Geophysical Fluid Dynamics Group).
The importance of understanding how faults respond to sources of fluids has been growing in the past years, not just because of scientific interest, but also due to societal concerns. For example, geothermal energy and oil-gas production can involve the injection of fluids in the underground that can occasionally produce damaging earthquakes. In this context, understanding the role of fluid processes is an important task. Fluids can highly affect the seismicity in natural fault zones, such as along megathrust faults in subduction zones or in strike slip fault zones, where the whole spectrum of seismicity can be observed from seismic slip producing regular detectable earthquakes, to slow slip events to aseismic creep, which can be observed based on field geology data and also from radar data. The transportation of fluids in faults zones can be strongly affected by the mechanical properties of the rocks. Pore fluid pressure is a parameter that can control the hydro-mechanical heterogeneity of faults. Fluid pressure variations can lead to their destabilization, affecting the seismic cycle. In 1992, Sibson proposed a link between stress exerted by the tectonic loading and variation of pore pressure. He proposed a fault-valve behavior that describes earthquake cycles as systems where pore pressure increases within the fault zone during tectonic loading, and decreases at the time of the earthquakes.
Understanding the evolution of pore fluid pressure during seismic cycles is therefore a crucial step to have more realistic seismic cycle models! If you are still here, let’s dig deeper into the subject!
What is new in our approach?
Many studies have been carried out in the past decades, to understand the processes resulting from the interaction between solids and fluids with rocks of different rheologies along natural fault zones (e.g. Van Dinther et al (2013). These studies address the importance of porosity evolution, and the (de)compaction of the complex visco-elasto-plastic porous media, once more highlighting the importance of fluid transport along the fault surface as well as the propagation of pressure waves in the fluid-filled porous media. To investigate this complex interaction on the seismicity, we choose to use a modified version of the recently proposed continuum based, fully-coupled solid-fluid approach from Petrini et al., (2020), which takes into account a realistic rheology of the poro-visco-elasto-plastic rock, where seismic events can propagate and form seismic cycles due to pore fluid pressure variations.
Why is our code different from others?
A Seismo-Hydro-Mechanical approach has been proposed recently (SHM; Petrini et al. 2020), which simulates seismic cycles by coupling inertia, rock strength and rock deformation with fluid flow in a single framework. This approach demonstrates that seismicity can be induced by fluid pressure variations (eq. Figure 1 right) without the incorporation of the widely used Rate-and-State dependent Friction law (RSF; Figure 1 left). RSF is often used to model seismicity along fault zones: the frictional weakening of the rock depends on the slip rate over the fault and on the contact properties within the sliding area. It also keeps a “memory of the deformation” using the state variable. The parameters are empirically determined based on laboratory experiments to approximate the variation of frictional shear strength. RSF builds up from two parts: (1) a direct effect, when the shear strength increases due to the increased slip rate; (2) an evolutionary effect, where the friction decreases, and the frictional instability leads to dynamic rupture.
In our latest publication (Dal Zilio et al. 2022a) we simulate realistic hydro-mechanical earthquake cycles in the context of a strike-slip fault zone, in a fully compressible medium with Rate-dependent strength (Yi et al. 2018) (RS; Figure 1 right). Although RS is similar to the direct effect of RSF (1), the evolutionary effect (2) is implemented differently: it is controlled by the local pressure changes and the poro-visco-elasto-plastic compaction of the medium, that will determine the shear strength of the rock once included the Drucker-Prager yield criterion. In this study, we have also tested many parameters (permeability, porosity, fluid- and solid-compressibility, Figure 3) to explore their influence on seismic cycles and slip modes.
Figure 1 : Comparison between the evolution of the state variable from Rate-and-state friction (left) and the effective pressure of our Rate-dependent strength approach (right). Plots show similar temporal variations illustrating the build-up and then the drop in stress during a seismic event.
Finally, how the changes in fluid pressure can cause dynamic slip in fault zones?
The results of our study indicate that pore fluid pressure variations only can lead to dynamic slip, and to seismic cycles! A seismic cycle builds up from 3 stages (Figure 2): (1) during the tectonic loading (also called inter-seismic period) the fault is loaded, and the visco-plastic compaction of the fluid-filled porous media occurs, which leads to the increase of the pore fluid pressure. (2) During the co-seismic phase, the increased pore fluid pressure lowers the shear strength of the rock, resulting in a shear instability, which causes the nucleation of the fluid-driven seismic event. The dynamic rupture propagates in the form of a pulse-like pressure wave at seismic speed. When the entire fracture energy is released, the propagation stops. (3) During the post-seismic phase, the pore fluid pressure is released and relaxes with time due to the elastic (de)compaction of pores. Then the fault can recover its higher strength, the stress can build up again, and the cycle can restart.
Figure 2 : Time step vs time plot and schematic pore and matrix deformation the during pre-, co- and post-seismic phases (modified from Petrini et al. 2020).
From our sensitivity analysis, we infer that the permeability and porosity highly influence the observed slip modes ranging from regular earthquake to slow slip events to stable creep. For example, an increase from 1% (Figure 3, left) to 10% (Figure 3, right) porosity, the occurrence of slow slip (Figure 3 in red) events is more likely. Whereas changes in permeability will promote the transition from aseismic creep to seismic events.
Figure 3 : Impact of solid-fluid compressibility on the slip pattern. (modified from Dal Zilio et al. 2022a)
Wow, you made it to the end, congrats! If you’re not bored to death and interested in learning more about our code and new approach, please contact me!
Behr, W. M., & Bürgmann, R. (2021). What’s down there? The structures, materials and environment of deep-seated slow slip and tremor. Philosophical Transactions of the Royal Society A, 379(2193), 20200218. Dal Zilio, L., Hegyi, B., Behr, W., & Gerya, T. (2022a). Hydro-mechanical earthquake cycles in a poro-visco-elasto-plastic fluid-bearing fault structure. Tectonophysics, 838, 229516 Dal Zilio, L., Lapusta, N., Avouac, J.-P., & Gerya, T. (2022b). Subduction earthquake sequences in a non-linear visco-elasto-plastic megathrust. Geophysical Journal International, 229 (2), 1098–1121. Petrini, C., Gerya, T., Yarushina, V., van Dinther, Y., Connolly, J., & Madonna, C. (2020). Seismo-hydro-mechanical modelling of the seismic cycle: Methodology and implications for subduction zone seismicity. Tectonophysics, 791, 228504. Sibson, R. (1992). Implications of fault-valve behaviour for rupture nucleation and recurrence. Tectonophysics, 211 (1-4), 283–293. Van Dinther, Y., Gerya, T., Dalguer, L., Mai, P. M., Morra, G., & Giardini, D. (2013a). The seismic cycle at subduction thrusts: Insights from seismo-thermo-mechanical models. Journal of Geophysical Research: Solid Earth, 118 (12), 6183–6202. Yi, J. T., Li, Y. P., Bai, S., Fu, Y., Lee, F. H., & Zhang, X. Y. (2018). A simple effective stress approach for modeling rate-dependent strength of soft clay. Journal of Offshore Mechanics and Arctic Engineering, 140 (4).