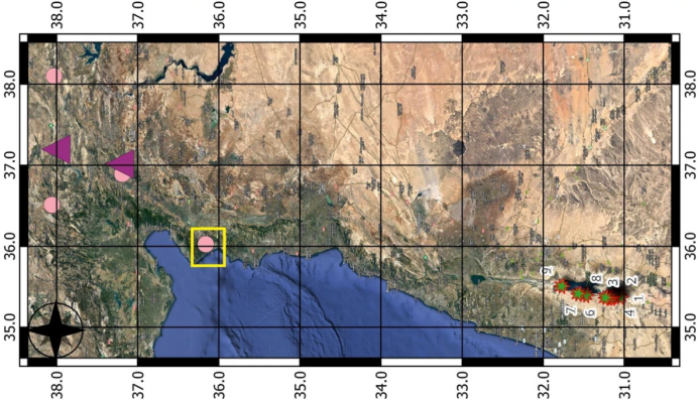
In this week’s blog, Shreeja Das, a Post Doctoral researcher at the Sami Shamoon College of Engineering, Ashdod, Israel working with Dr. Vladimir Frid, discusses her research involving the use of FEMR waves and its use as a tool in predicting impending geohazards , some of her results obtained using said technique to study transform fault activity along the Dead Sea Transform fault.
Earthquakes have long been a source of fascination, dread, and curiosity. One of the longest-standing questions in the field of seismology and geohazard management has been our ability to predict upcoming seismic or faulting events. While many other geophysical, geological, and geochemical proxies are invoked when trying to predict the probability of large-scale faulting events, the formation and propagation of Fracture-Induced Electromagnetic Radiation (FEMR) has emerged as a prolific geophysical tool, offering valuable insights into geohazards. FEMR operates on detecting electromagnetic radiation generated by rocks experiencing differential stresses and undergoing brittle deformation within the Earth’s near-surface crust. Under external forces, such as those in thrust or shear zones, these rocks develop microcracks that propagate, emitting FEMR waves typically in the kHz to MHz frequency range (Bahat, 2005, Frid et al., 2003, Rabinovitch et al., 2007).
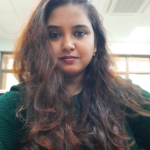
Shreeja Das is currently a postdoctoral researcher working on several applications of the novel technique of FEMR .
The intensity of FEMR pulses correlates with the degree of stress accumulation, indicating a potential macro failure. Conversely, as microcracks coalesce and lead to macro failure, the amplitude and frequency of FEMR pulses decrease. This phenomenon often warns of impending macro failures, sometimes hours or days before their occurrence. Given FEMR’s lower attenuation compared to seismic waves, it presents a more efficient precursor to macro failure events. Moreover, being a non-contact and non-destructive technique, data can be procured relatively quickly, easily, and economically compared to conventional methods.
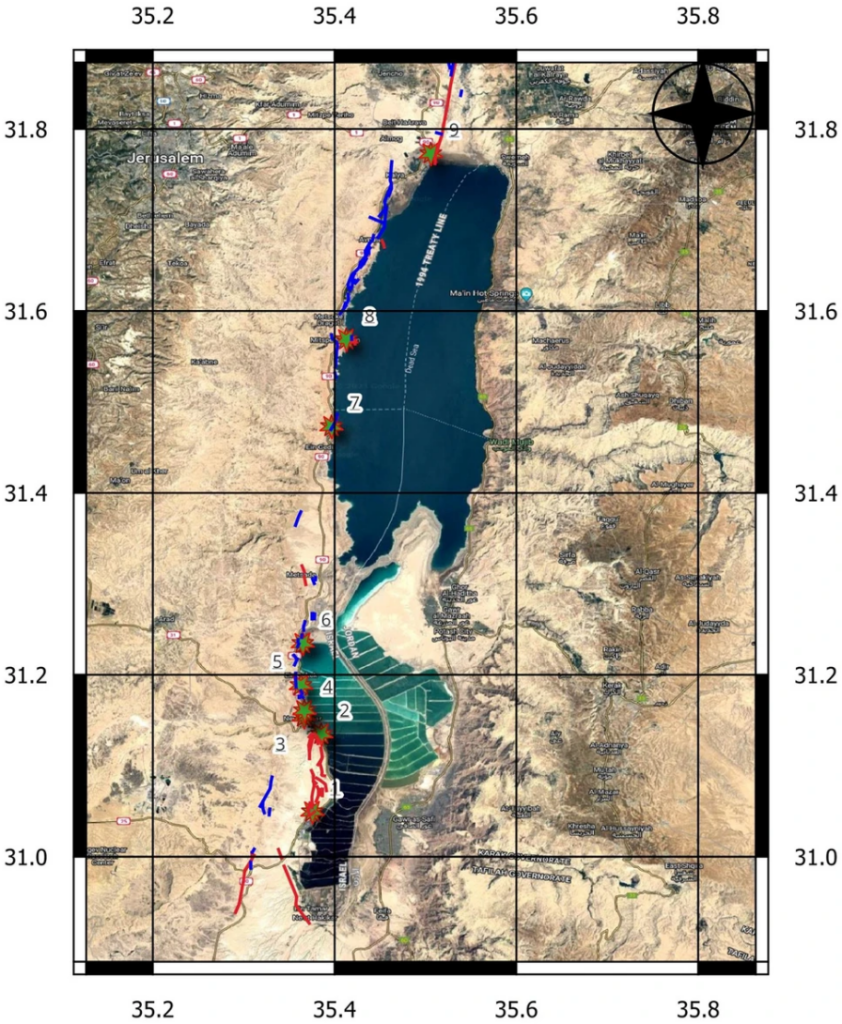
The map of the Dead Sea basin with the superimposed location of FEMR monitoring stations (green stars and numbers). The red and blue lines indicate the location of active and potentially active faults, respectively (Image taken from Das et al., 2024).
The Dead Sea fault is a transform boundary that periodically experiences moderate to large magnitude earthquakes due to the strike-slip transform boundary between the African plate to the west and the Arabian plate to the east. We carried out FEMR surveys at 9 locations along the Dead Sea Basin. Incidentally, these surveys were conducted a couple of hours before the occurrence of a 6.4 magnitude earthquake which was reported along the Syrian-Turkish border (Dated: 20/02/2023). In the context of our studies, the timing of the earthquake was fortuitous as it aided in assessing the trends displayed by FEMR parameters such as FEMR activity (‘hits’), Benioff Strain release, Energy, Amplitude, and their associated crack dimensions leading up to the earthquake.
The survey was conducted using a portable ANGEL-M device which enables the acquisition of the FEMR. It consists of three pluggable ferrite antennas, a microprocessor-controlled receiver unit, an analog and digital processor unit, an analog-digital converter, and a power supply unit along with a few other essential components such as a RAM and interface card to store the raw data. The receiver’s front panel contains an LCD and a 10-pin multi-functional connector designed to connect the receiver to a PC, antenna, or charger. The instrument can measure a range of frequencies between 5-150 kHz and has a sensitivity of 1 mV/m. At each location, the three antennas are oriented in a mutually orthogonal direction to each other, and measurements are usually taken for 10 seconds. The collected raw data is then filtered using the ANGELWORKS software in order to eliminate noise.
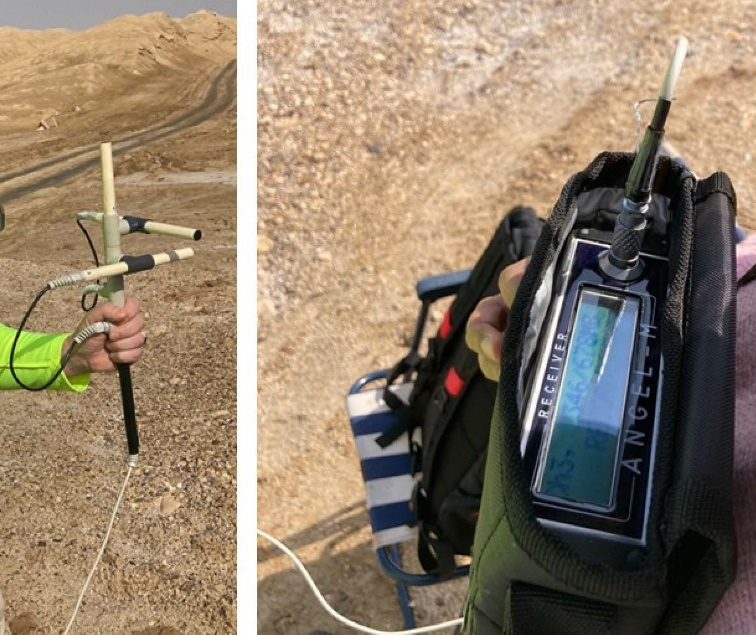
The photographs of the 3D antenna and Angel-M instrument during measurements at the Dead Sea region (image from Das et al., 2024).
Some of the distinctive results obtained during the study will be described here. We constructed a plot showing the cumulative FEMR amplitude (TDBS) and FEMR hits over time before an earthquake. The time axis represents the duration between data recording and the earthquake occurrence. Three stages of the Benioff strain are identified, which are correlated to the different stages of nucleation. During the first stage, there is a gradual increase in the slope of the TDBS, representing the early nucleation stage associated with a steady increase in stress levels. The second stage is characterized by a steep rise in the TDBS slope, indicating the accelerated stage where more new minor cracks are formed. The slope of the TDBS falls back to a steady increase with smaller differential changes in cumulative amplitude or dynamic propagation stage. This is related to a state of irreversibility where the gradual and steady slope indicates that with an increase of time, t.
Comparing the evolution of the FEMR hits over the same range of time will show us if there is any correlation between the evolution of the Benioff strain and the amount of radiation emitted during FEMR. As we can see, FEMR hits show increasing activity temporally (toward the earthquake) and the cyclic trend in FEMR hits indicates alternating periods of high activity and silence, potentially linked to stress changes during crack propagation. Comparing the crack length (black line), crack width (olive line), and crack area (purple line) vs the FEMR hits chart – the green line, shows that in the 3rd stage, the crack area (a relevant 1st order contribution to the crack dimensions) anomalously increases indicating an impending failure. This study provides crucial insights into the analysis of FEMR parameters and their patterns before earthquakes. One limitation of this study is that since the seismic event took place shortly after the survey, the effect of stress saturation is something we could not further investigate.
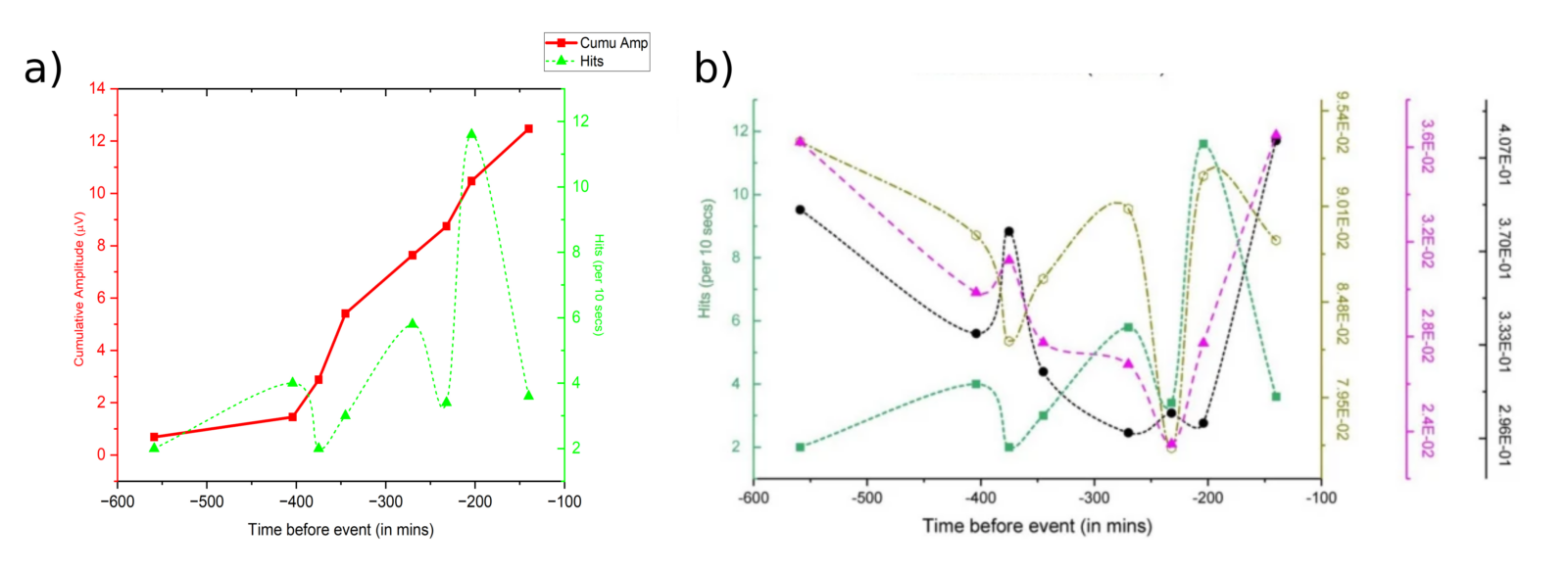
a) The chart of cumulative FEMR amplitude (the “Benioff strain release diagram” TDBS) – red curve and FEMR Hits– green curve versus the time before the earthquake event. b) The plot for crack length (black line, m), crack width (olive line, m), crack area (purple line, m2), and FEMR Hits (image from Das et al., 2024).
Currently, a significant application of this technique is in the mining industry where FEMR can be prolifically used to delineate zones of “accumulated stresses” which may indicate zones vulnerable to rock bursts or roof falls in the future. The technique of FEMR has gained an increasing amount of impetus in the past decade due to its vivid contributions to large-scale investigations such as earthquakes and landslide forecasting. Although several FEMR studies have previously been conducted in copper mines and coal mines, its potential as a tool for predicting rock burst hazards and assessing geological instability in the mines remains under-explored. After delineating the specific zones in the mines where stresses accumulate, it can be complemented with standard stress-relieving techniques, such as blasting or drilling. This would, in turn, aid in building a state-of-the-art early warning system network utilizing the FEMR technique to predict the occurrence of geohazards such as rock bursts or roof falls.
References Bahat, D., Rabinovitch, A., Frid, V. (2005). Tensile fracturing in rocks-tectonofractographic and electromagnetic methods. XIV, Springer, Heidelberg, pp.570. Das, S., Frid, V., Rabinovitch, A., Bahat, D., & Kushnir, U. (2024). Insights into the Dead Sea Transform Activity through the study of fracture-induced electromagnetic radiation (FEMR) signals before the Syrian-Turkey earthquake (Mw-6.3) on 20.2. 2024. Scientific Reports, 14(1), 4579. Frid, V., Rabinovitch, A., & Bahat, D. (2003). Fracture induced electromagnetic radiation. Journal of physics D: applied physics, 36(13), 1620. https://doi.org/ 10.1088/0022- 3727/36/13/330 Frid, V., Wang, E. Y., Mulev, S. N., & Li, D. X. (2021). The fracture induced electromagnetic radiation: Approach and protocol for the stress state assessment for mining. Geotechnical and Geological Engineering, 39, 3285-3291. Rabinovitch, A., Frid, V., Bahat, D. (2007). Surface oscillations—a possible source of fracture-induced electromagnetic radiation. Tectonophysics, 431,15–21.