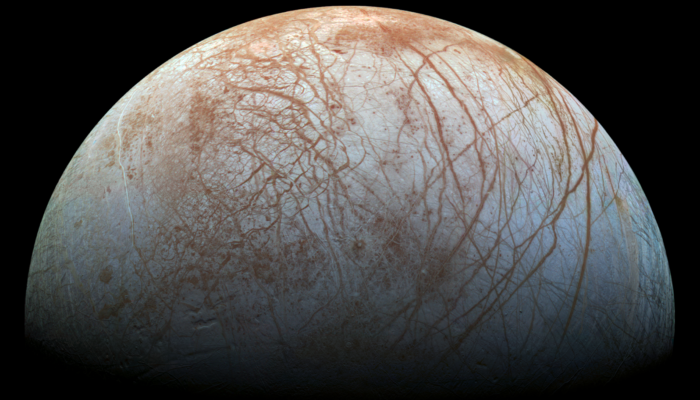
“Europa may be one of the most promising places in our solar system to find present-day environments suitable for some form of life beyond Earth.” This week in News & Views, Kevin Trinh, a PhD student at Arizona State University, discusses how his recent works on Jupiter’s moon Europa can enlighten us about the evolution of Europa’s interior.
One of the most promising places to search for alien life is Jupiter’s moon, Europa (e.g., Chyba and Phillips, 2002). Underneath Europa’s icy surface is a salty ocean of liquid water (e.g., Kivelson et al., 2000). The evidence of the ocean is compelling. In the late 1990s, the NASA Galileo spacecraft flew by Europa several times with a magnetometer — an instrument that measures magnetic fields. Europa does not have its own intrinsic magnetic field; Instead, Europa produces an induced magnetic field, which is a conductor’s response to an external (i.e., Jupiter’s) magnetic field. The best explanation for Europa’s induced magnetic field is a global salt water ocean.
In addition to liquid water, life as we know it requires an energy source and a suite of biologically essential elements (e.g., Hand et al., 2020). For over 20 years, many studies assumed that Europa consists of four layers—an ice shell, a salty ocean, a rocky mantle, and an iron-rich core at the center (e.g., Anderson et al., 1998)—on the basis of Galileo spacecraft gravity measurements. Challengingly, gravity-constrained models suffer from ambiguity, scientists need to assume the number of layers within a planetary body before calculating the plausible thickness and density of each layer.
Differentiation, the formation of layers inside a planet, requires that we melt enough of the interior for the densest components to migrate towards the planetary center. For large rocky planets, it is reasonable to assume that a metal core formed during accretion—the formation of the planet itself—but small planetary bodies may not form with sufficient energy to differentiate the rock and metal from each other. Europa totals to a meager 1% of Earth’s mass, so scientists needed to re-evaluate the likely starting conditions for a small body like Europa.
Recently, Trinh et al. (2023) published a paper in Science Advances about the formation and slow evolution of Europa. Instead of being born straight into adulthood, Europa could have experienced an eventful life where its interior differentiates twice. A small moon like Europa may have started as a cold mudball. Over time, as radioactive isotopes and tidal forces produce heat, Europa may melt ice or warm up the rock to produce the ocean and ice shell (e.g., water-rock differentiation). Later, as temperatures continue to rise, rock and metal may differentiate at even higher temperatures (> 1250 K). The timing of metallic core formation depends on many physical unknowns, but the common result is that Europa formed its metallic core billions of years after accretion, if at all. The results of Trinh et al. (2023) are a stark contrast to other studies that assumed early metallic core formation at Europa.
Excitingly, the NASA Europa Clipper and ESA JUICE missions will arrive at the Jupiter system in the years 2030 and 2031, respectively. NASA Europa Clipper will focus exclusively on Europa with over 40 planned fly-bys around the moon. On the other hand, JUICE will visit the three icy Galilean moons of Jupiter, which includes Europa. Collectively, these missions may help test models of Europa’s interior structure, which is a critical step in evaluating the moon’s astrobiological potential.
References: Anderson, J. D., Schubert, G., Jacobson, R. A., Lau, E. L., Moore, W. B., & Sjogren, W. L. (1998). Europa's differentiated internal structure: Inferences from four Galileo encounters. Science, 281(5385), 2019-2022. Chyba, C. F., & Phillips, C. B. (2002). Europa as an abode of life. Origins of Life and Evolution of the Biosphere, 32, 47-67. Hand, K. P., Berisford, D., Daimaru, T., Foster, J., Hofmann, A. E., & Furst, B. (2020). Penitente formation is unlikely on Europa. Nature Geoscience, 13(1), 17-19. Kivelson, M. G., Khurana, K. K., Russell, C. T., Volwerk, M., Walker, R. J., & Zimmer, C. (2000). Galileo magnetometer measurements: A stronger case for a subsurface ocean at Europa. Science, 289(5483), 1340-1343. Trinh, K. T., Bierson, C. J., & O’Rourke, J. G. (2023). Slow evolution of Europa’s interior: metamorphic ocean origin, delayed metallic core formation, and limited seafloor volcanism. Science Advances, 9(24), eadf3955.