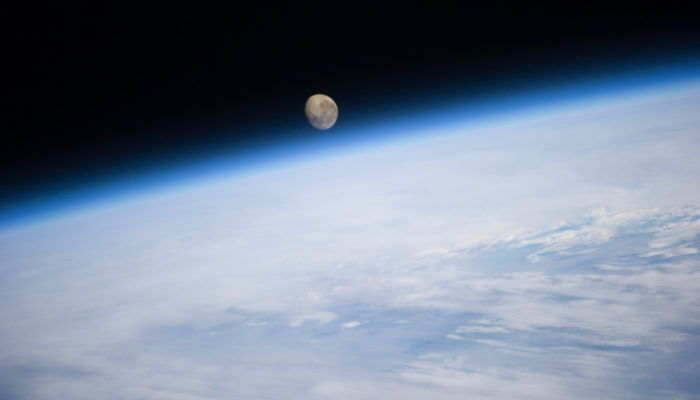
This week on the GD Blog we are taking a magical geodynamicist’s mystery tour to our planet’s Moon thanks to Tobias Rolf, Researcher at the Centre for Earth Evolution and Dynamics (CEED) at the University of Oslo, Norway (currently a Visiting Researcher at the Institute of Geophysics at the University of Münster, Germany).
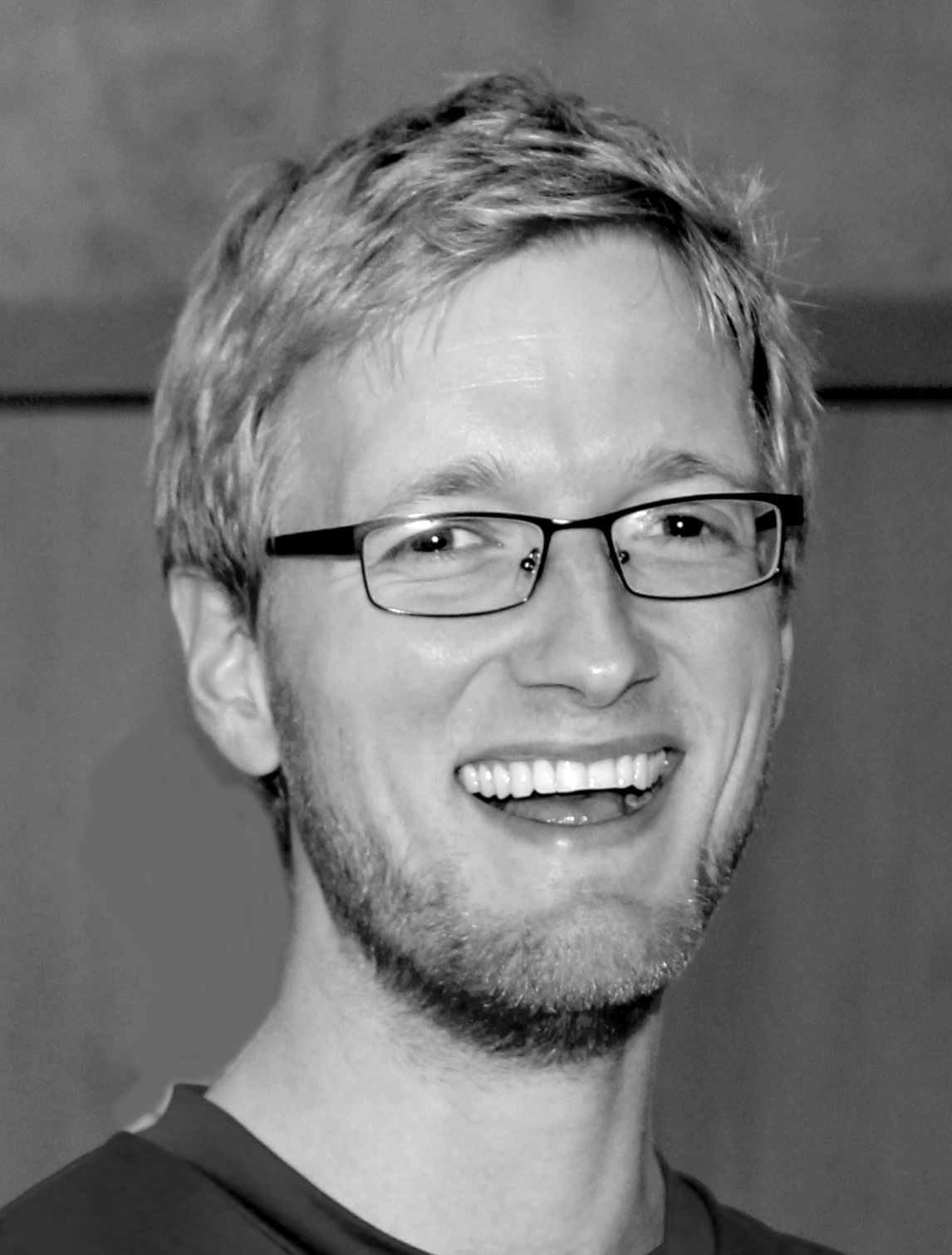
Tobias Rolf (CEED, U.Oslo)
Imagine you are orbiting the Earth at an altitude of a few hundreds of kilometres. What you see is rather impressive: large continents, even larger oceans, polar ice caps, huge cloud patterns. If timing is in your favour you can even see structures made by our own prolific two-legged species (the Great Wall of China, for example!). In short, you see an exciting manifold of structures and colour and, as the geodynamicists we are, our curiosity is immediately peaked about what causes these phenomena.
Now turn around. What do you see? Nothing, except some pitch black punctuated by little stars hanging out in the distance? Could be, but if timing is again in your favour, you might see something else, not too far in front of you. Yes, that’s the Moon. Do you remember the colours and beauty we have just seen in the other direction? You surely do, but what do you see now, the same? No, what you look at seems like a rather monotone rocky body. Very grey, no oceans, no cloud patterns. Not so exciting, plus, rather small. You feel this urge to turn around and look back at the Earth, but hey, you would not want to be dismissed because of colour, size and look, would you? So, let’s have a closer inspection.
Today’s most accepted scenario of the Moon’s formation is the collision of a giant impactor with the very young Earth. This ripped off a substantial portion of the Earth’s proto-material and ejected it into space where it accumulated into another planetary-scale object at a distance of (today!) ~380 000 km away from Earth. This all happened around 4.5 billion years ago and, since then, the Earth and Moon formed a joint system (remember what causes the tides on Earth, for instance?). Both share, at least partly, the same basic compounds.
At this point, any similarity seems over. On Earth, we see these exciting expressions of mantle dynamics and plate tectonics going-on. On the Moon, however, we see a lot of fine dust that covers much of the planet, it looks rather dry and dead. What we do see though, are craters, a lot of them. Modern spacecraft missions provide us with super-high resolution images of the lunar surface, so we can really see the whole variety of these mostly circular structures. We can also make statistics about their frequency in certain areas, which tells us about how old they are with respect to other regions. Some smaller craters appear as almost perfect bowls, while others feature rings, peaks, terraces, and mounts inside them. Models of impact crater formation [1] tell us how they may form and from the different morphologies we can learn a lot about the structure and properties of the lunar surface [2].
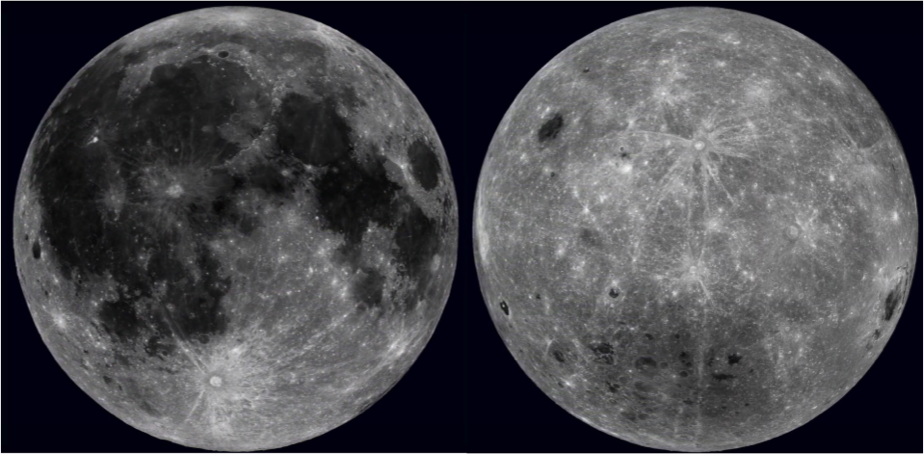
Figure 1: Near-side (left) and far-side (right) of the Moon. Both images were taken by cameras aboard the Lunar Reconnaissance Orbiter spacecraft. Circular features indicate impact structures. The dark-shaded zones mostly correlate with basaltic volcanism filling the near-side impact basins. Image Credit: NASA/Goddard Space Flight Center/Arizona State University, published on October 20, 2017 on https://moon.nasa.gov/resources/
And there are the big guys, the basins. These are typically defined as those impact craters with a diameter of more than 200-300 km, but there are some that exceed even a 1000 km. You would need a huge impactor to create one of these, and their impact must really have shaken the Moon quite a bit. Don’t worry, this has been very rare and the chances for repetition today are rather slim! Such large events deliver a lot of energy to the target: part of it is used to create the basin, to displace material, and to eject it out from its initial location to a potentially far away location. Another part is released as heat within the target. This can excite melting in the interior and initiate volcanism, which is why these basins are often filled with basaltic lava flows that creates the large dark-shaded areas, the mare, which we can easily see from the Earth’s surface (Figure 1). At least on the lunar near-side, that is, the face of the Moon is shown to us permanently because it is tidally-locked. On the far-side, basins are generally smaller and fewer basaltic flows are present [3].
We think this is due to a hemispheric asymmetry in the Moon’s interior structure with the near-side being hotter and the far-side being colder (also, the thickness of primordial crust is larger on the far-side). After its formation, the Moon was still very hot and contained a magma ocean, but this did not cool quite uniformly. Instead, the magma ocean persisted slightly longer on the nearside and this focused much of the incompatible, heat-producing elements into this hemisphere [4] to maintain warmer conditions even on the long-term. A-ha! So the initially dead and a little dull looking surface of the small Moon tells us a lot about the interior structure and about magma ocean cooling – a process that definitely shaped the early Earth and its subsequent evolution as well!
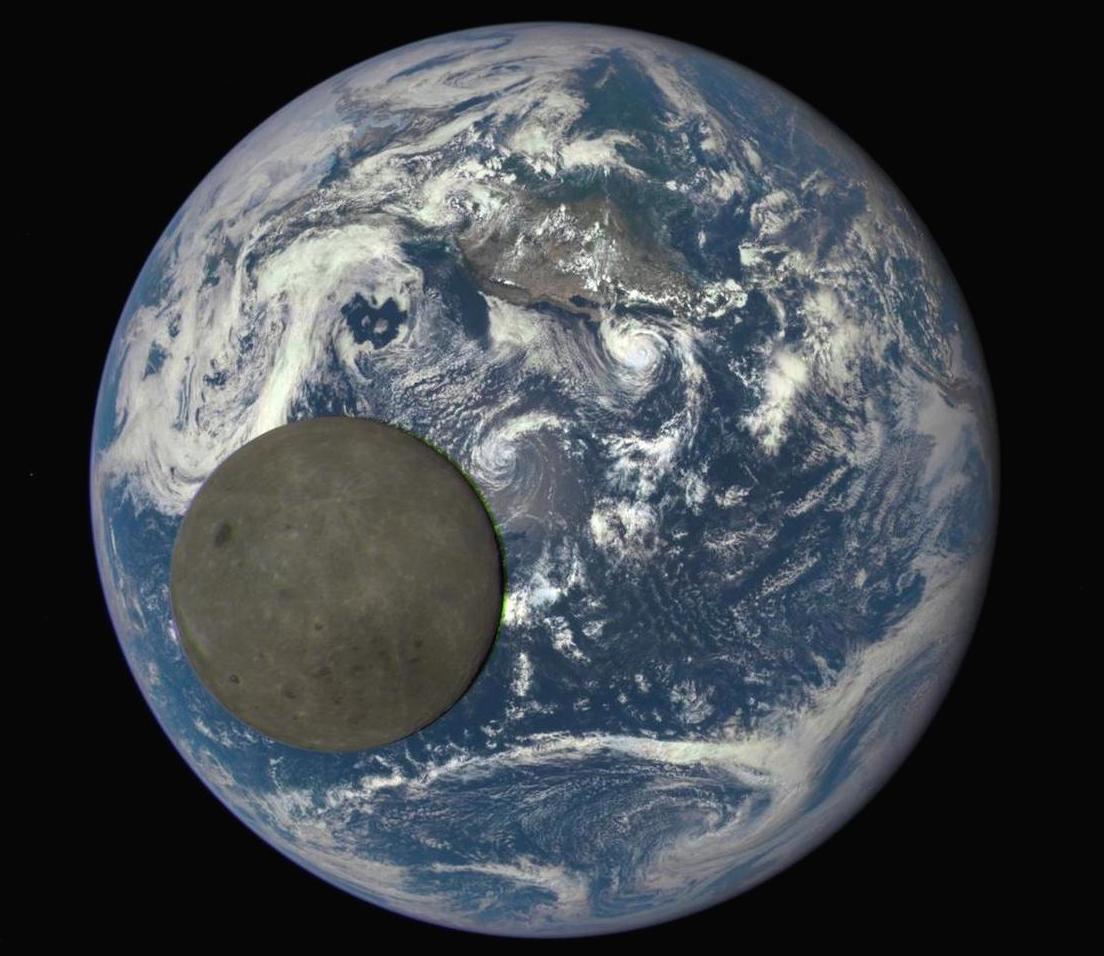
This unique image shows the far side of the moon, illuminated by the sun, as it crosses between the DISCOVR spacecraft’s Earth Polychromatic Imaging Camera (EPIC) camera and telescope, and the Earth – one million miles away. Credits: NASA/NOAA
But let’s go back to the surface of the Moon, where all of these rocks and fragments lie around, having been ejected from some nearby basins. The fantastic thing about the Moon is that we have samples! They come from meteorites, but also from sample returns like during the Apollo missions. In the lab, we can determine their ages, which provides the cornerstones of the lunar chronology. Of course, this remains an almost overwhelming puzzle as it is not easy to link the sample to a particular location; it could have its origin exactly where we picked it up, but perhaps it was deposited there as ejecta from a huge basin-forming impact elsewhere instead. Deciphering this is very hard and conclusions are often controversial, but it is important. Resolving this tells us when the large impacts on the Moon occurred, even in absolute terms. Based on this, we can estimate how the frequency of impacts (the impactor flux) has evolved with time and so we have a key in our hands to decipher the cratering record of other planetary bodies (linked to the same, possibly rescaled, impactor flux). Oh, and while we are at it, the Moon can therefore even tell us a lot about solar system time scales and history – something that the Earth can hardly do, because plate tectonics has wiped out any record of this.
In conclusion, the Moon may look small and, geodynamically at least, a little dull and insignificant. However, always remember that it is one of the most precious archives we have. Understand it and you understand not only the Moon, but also the evolution of the (early) Earth and the entire solar system a lot better.
Keep looking at it (and not only the Earth)!
References [1] iSALE code: http://www.isale-code.de, a community code for modelling impact cratering processes, developed by G. Collins, K. Wünnemann, D. Elbeshausen, T. Davison, B. Ivanov, and J. Melosh (amongst others) [2] Prieur, N., Rolf, T., Wünnemann, K., Werner, S.C., 2018, Formation of simple impact craters in layered targets: implications for lunar crater morphology and regolith thickness, J. Geophys. Res. Planets, 123, 1555-1578, DOI:10.1029/2017JE005463 [3] Miljkovic, K., Wieczorek, M.A., Collins, G.S., Laneuville, M., Neumann, G.A., Melosh, H.J., Solomon, S.C., Phillips, R.J., Smith, D.E., Zuber, M.T., 2013, Asymmetric Distribution of Lunar Impact Basins Caused by Variations in Target Properties, Science, 342, 724-726, DOI:10.1126/science.1243224. [4] Parmentier, E.M., Zhong, S., Zuber, M.T., 2002, Gravitational differentiation due to initial chemical stratification: origin of lunar asymmetry by the creep of dense KREEP?, Earth Planet. Sci. Lett., 201, 473-480, DOI:10.1016/S0012-821X(02)00726-4