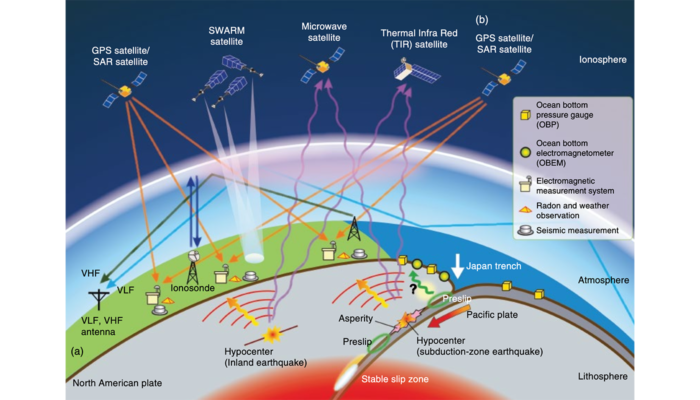
The fundamental of Space Geodesy is the observation of the dynamics of the Earth, such as its rotation on its axis, changes in shape, and the external gravitational field etc., which allow for the monitoring of the Earth system in general. Space Geodetic techniques, such as Global Navigation Satellite Systems (GNSS) / Global Positioning System (GPS), as well as Interferometric Synthetic Aperture Radar (InSAR), can be used to measure the displacement of the Earth’s lithosphere and its interaction/energy exchange with the surrounding atmosphere very accurately. Past two decades, Space Geodetic observations in terms of tectonic crustal deformation and ionospheric electron perturbations have substantially improved. In this week’s News and Views, we have Dr. Sunil P. S. discussing some of the Lithosphere-Atmosphere-Ionosphere coupling studies by their research group in the Department of Marine Geology and Geophysics, Cochin University of Science and Technology (CUSAT), Kochi, India in collaboration with different national and international research groups
An earthquake occurs, when tectonic strain builds up throughout the interseismic period to a point where friction on the fault can no longer support the stress. A net translation of the material on either side of the fault results from the recovery of the long-term accumulated elastic strain during the coseismic event. This unexpected release of strain has two significant and observable effects. First, the energy released causes a brief excitation of elastic waves, which can cause structural damage to buildings because they travel into the Earth as body waves or along the free surface as surface waves. Seismometers can detect these waves anywhere in the world, and they can be used to alert people to possible impacts. Second, the permanent displacement of the surface with respect to near and far-field can be determined using techniques like GNSS and InSAR.
When an earthquake triggers, the Earth’s lithosphere and its surrounding atmosphere function as a coupled system, influencing one another through several coupling mechanisms in response to various phenomena such as active tectonics, earthquake deformation, tsunami propagation, volcanic eruption etc. The exchange of energy at the boundary between lithosphere and atmosphere is very small due to the significant contrast in acoustic impedance. During large magnitude earthquakes/tsunami associated with any active tectonics, the impulsive ground motion or water column movement near the epicentre introduces acoustic waves in the overlying atmosphere. These waves then propagate to the ionospheric altitudes and cause perturbations in the electron density, which are generally referred as Co-seismic Ionospheric Perturbations (CIPs). Various ionospheric sounding techniques such as ionosonde, Total Electron Content (TEC) measurements using Global Navigation Satellite Systems (GNSS), HF Doppler radar, in-situ observations from low Earth orbiting satellites, airglow imager-based observations, etc. are used to study the characteristics of CIP. However, in recent years, the GNSS-TEC observations are widely used in this field because of its large spatial and temporal coverage.
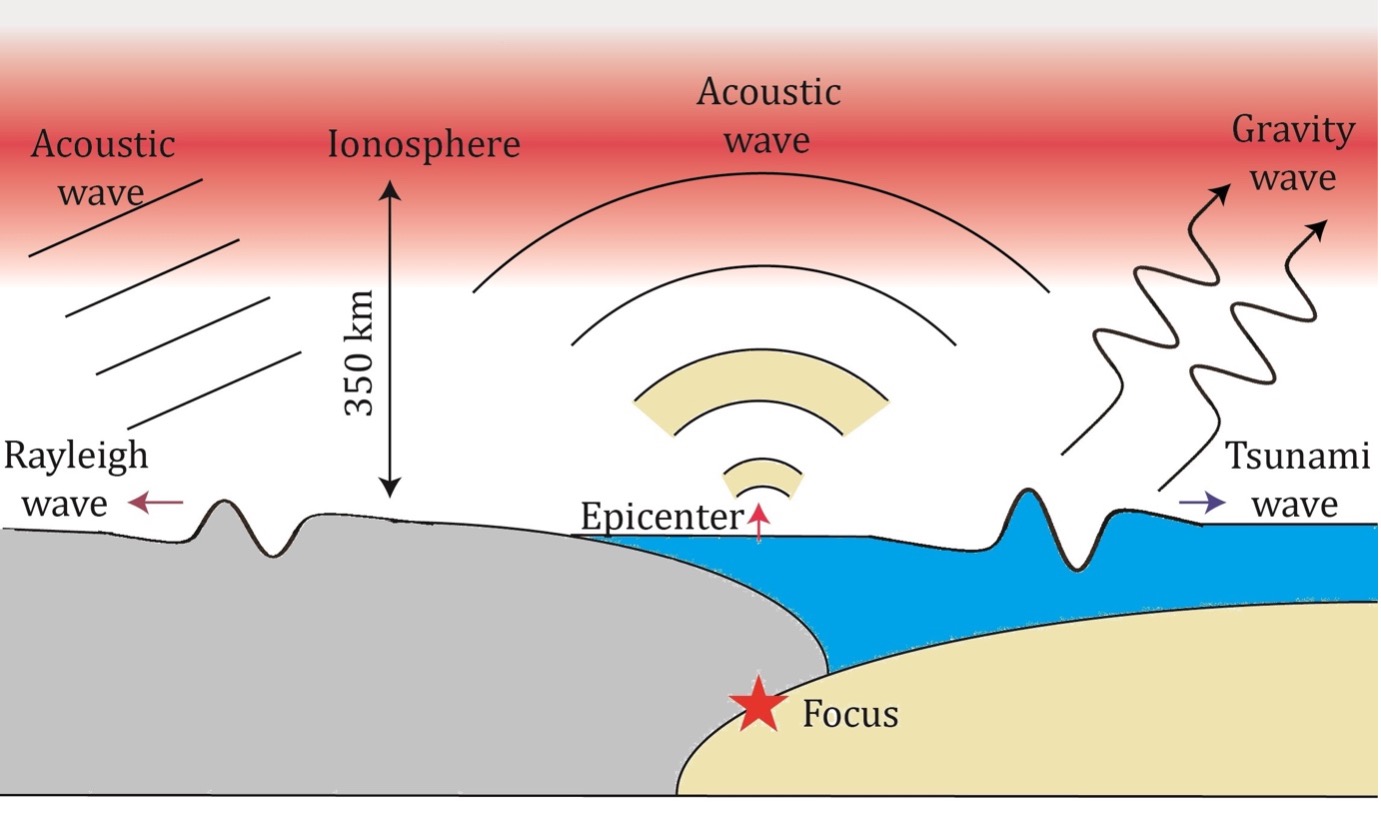
Figure 1: Schematic diagram illustrating the lithosphere-atmosphere-ionosphere coupled system. The impulsive co-seismic ground motion introduces acoustic waves in the overlying atmosphere near the epicenter. Away from the epicenter, the ground motion due to Rayleigh waves generate acoustic waves. During underwater earthquakes, tsunami waves in oceans generate gravity waves in the atmosphere.
1. Interseismic Lithospheric Deformation
For a better knowledge of the state of strain accumulation around the plate boundaries and active faults, crustal deformation monitoring is essential. Nowadays, high temporal resolution recordings of crustal deformation are normally made using the GNSS and InSAR data sets. To study the interseismic strain accumulation associated with 25 April 2015, Mw 7.8 Nepal earthquake we have used both continuously observed GNSS and InSAR data sets from Nepal Himalayan regions (Sreejith et al., 2018). The interseismic deformation study revealed that the 2015 earthquake ruptured an asperity that had been subject to substantial strain and stress accumulation before the incident. The study also observed that a comparable asperity with retained strain energy is found to the west of the Gorkha event’s epicentre. It is suggested that this could trigger an earthquake of similar size to the one that struck Gorkha, Nepal, in 2015.
2. Earthquake triggered ground deformation and ionospheric perturbation
Many comprehensive investigations have been performed to deduce earthquake source parameters (such as crustal uplift/subsidence, rupture propagation etc.) from CIPs. However, the CIP characteristics could not be directly linked to tectonic forcing because they are not the only factor to play. Other parameters, such as geomagnetic field, GNSS satellite observation geometry, ionospheric background electron density, can considerably influence the growth of CIP at ionospheric altitudes. In a recent study (Sunil et al., 2022), a detailed investigation of subsurface, surface, and ionospheric characteristics associated with the Mw 7.8 Chignik Earthquake, Alaska is conducted. Using continuous Global Positioning System (cGPS) inverted co-seismic displacements, it has been shown that the earthquake slip is distributed along the rupture area northeast of the epicentre. The vertical surface displacement estimated from the co-seismic slip shows a similar pattern with a maximum uplift of around 1 metre occurring over the high slip area. To investigate the characteristics of ionospheric imprints of this event, cGPS-derived TEC data was used. In contrast to the north-east directed co-seismic rupture and surface uplift, the ionospheric signatures of this event were found to be more significant southwest of the epicentre. This study has revealed that the orientation of TEC perturbations was predominantly controlled by Earth’s magnetic field and the background density of the ionosphere, rather than the propagation of rupture and co-seismic uplift pattern.
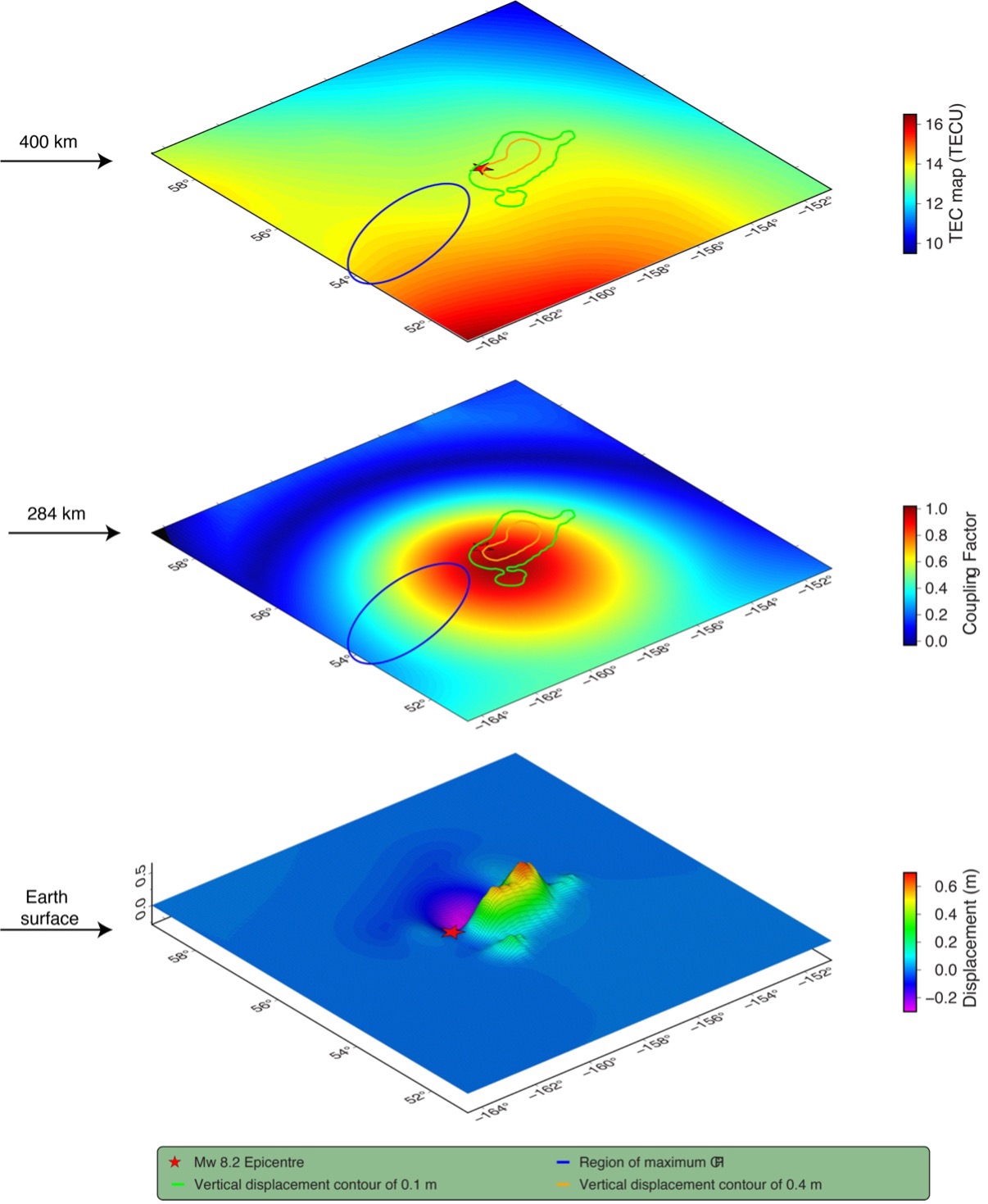
Figure 2: The 3D representation of co-seismic vertical displacement, geomagnetic coupling factor and background TEC map respectively from bottom to top associated with the 29 July 2021, Mw 8.2 Chignik, Alaska earthquake. Red stars represent the earthquake epicentre, and the blue ellipse highlights the region of maximum CIP amplitude. Green and orange contours on the middle and top panel show the vertical displacements 0.1 m and 0.4 m respectively.
3. Tsunami detection using GPS-TEC
Tsunami early warning systems using the ionospheric signatures is a relatively new and evolving research area. The vertical movement of the water column during oceanic earthquakes generate acoustic-gravity waves in the overlying atmosphere and ionosphere. By continuously monitoring these signals in real-time, it is possible to develop a tsunami early warning system. We studied two Chilean tsunamigenic subduction earthquakes, the Mw 8.1 Pisagua earthquake in 2014 and the Mw 8.3 Illapel earthquake in 2015, to better understand the coupling between earthquake and tsunami using variations in the GPS derived ionospheric total electron content (GPS-TEC) (Shrivastava et al., 2021). The GPS-TEC changes were represented by three types of signals. The initial weaker signal was well correlated with the Acoustic Rayleigh wave, while the subsequent stronger perturbation was believed to be caused by the Acoustic Gravity wave and Internal Gravity wave, which were respectively induced by earthquakes and subsequent tsunamis. It is suggested that within a framework of multi-parameter early warning systems, GPS-TEC variations can be used to assess earthquake occurrence and tsunami propagation.
4. Volcanic induced atmospheric and ionospheric effects
Like earthquakes and tsunami, massive volcanic eruptions also generate significant atmospheric and ionospheric effects. These eruptions cause sudden injection of energy, mass, and momentum into the atmosphere, resulting in the generation of various atmospheric and ionospheric waves. In a recent study, different modes of atmospheric and ionospheric perturbations associated with the Hunga-Tonga volcanic eruption occurred on 15th January 2022 are investigated in detail (Ajith et al., 2022). Presence of highly convective zone and the existence of concentric Lamb and gravity waves at lower atmospheric altitudes are confirmed by the visible and infrared channel data from GOES-17 satellite and Atmospheric Infrared Sounder (AIRS) data from NASA’s aqua satellite. Moreover, TEC data from 175 GPS stations covering the entire New Zealand region are investigated to identify ionospheric electron density perturbations. The study revealed the existence of two dominant modes of Travelling Ionospheric Disturbances (TIDs) over the New Zealand region having periodicity between 30-50 minutes. These two distinct modes, with speeds of 542 m/s and 354 m/s, have been identified as being associated with atmospheric gravity waves and gravity waves triggered by Lamb waves, respectively. Both types of waves have been observed propagating away from the volcano in a south-west direction over the New Zealand region.
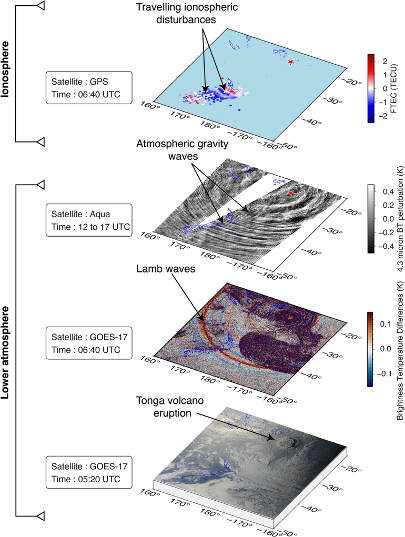
Figure 3: The signatures of the Tonga volcanic eruption recorded at lower atmospheric and ionospheric altitudes. (a) GOES-17 visible image; (b) IR Brightness temperature difference observed by GOES-17; (c) AIRS observations of stratospheric Gravity Waves; ( d) Travelling ionospheric Disturbances (TIDs) observed at ionospheric altitudes.
References
K.M. Sreejith, P.S. Sunil, Ritesh Agrawal, Ajish P. Saji, A.S. Rajawat, D.S. Ramesh (2018). Audit of stored strain energy and extent of future earthquake rupture in central Himalaya, Scientific Reports – Nature, Vol. 8:16697, DOI:10.1038/s41598-018-35025-y.
Sunil, A. S., Sunil, P. S., Shrivastava, M. N., Maurya, A. K., Thomas, D., & Gonzalez, G. (2022). Seismic induced ground deformation and ionospheric perturbations of the 29 July 2021, Mw 8.2 Chignik Earthquake, Alaska. Journal of Geophysical Research: Space Physics, 127, e2022JA030576. https://doi. org/10.1029/2022JA030576.
M.N. Shrivastava, A.K. Maurya, G. Gonzalez, P.S. Sunil, J. Gonzalez, P. Salazar, R. Aranguiz (2021), Tsunami detection by GPS-derived Total Electron Content, Scientific Reports – Nature, https://doi.org/10.1038/s41598-021-92479-3.
Ajith, K.K., Sunil, A.S., Sunil, P.S. et al. (2022). Atmospheric and Ionospheric Signatures Associated with the 15 January 2022 Cataclysmic Hunga-Tonga Volcanic Eruption: A Multi-layer Observation. Pure Appl. Geophys. 179, 4267–4277 (2022). https://doi.org/10.1007/s00024 022-03172-z.