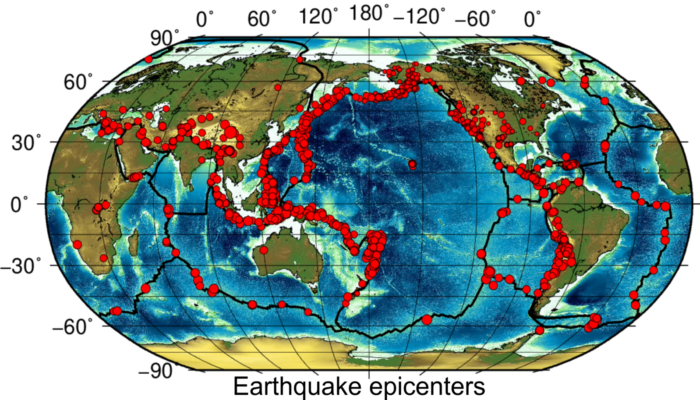
As fascinating as the plate tectonics and its accompanying dynamics are, continental interiors are no quiescent zones. The tectonics within these interiors are apparent in the occurrence of numerous intraplate earthquakes, and this week, EGU blog editor Arushi Saxena briefly discusses them and their mechanisms.
Earthquakes are a fairly common occurrence here on Earth’s crust. Although most are essentially imperceptible to the average human going about their business, several dozen earthquakes of Richter magnitude 2 or greater occur every day; The above figure shows a representative sample of potentially noticeable shakes that were recorded in the month prior to writing this article. It is worth mentioning that most of those events occur at plate boundaries, which are far away from where people live.
The mechanism of the interplate earthquakes is well understood in the geoscience community– collisions between adjacent tectonic plates build up energy before releasing it once the fault strength is exceeded. Somewhat harder to explain are the less-frequent earthquakes that occur in concentrated zones within the plate interior known as intraplate earthquakes. After all, it seems like there should be relatively little force exerted far from the boundary. Why should these seemingly insulated zones be at risk for accumulating stresses required to release a detectable (or even dangerous) earthquake? Understanding the origin of intraplate earthquakes is key to mitigating the seismic hazard to life and property they pose. Despite decades of research, the debate is still lively and some fundamental questions remain unanswered.
There are two schools of thought in models with regards to spatial limitations of earthquake-susceptible zones. The first, concentrated intraplate seismicity, argues that these intraplate seismic zones are a spatially limited phenomenon, and repeated intraplate earthquakes occur within an area while broader forces prevail over geological time spans (e.g., Thomas and Powell 2017). The second, migrating intraplate seismicity supports that intraplate seismic zones are somewhat random manifestations of transient stress perturbations, and once such a fault has ruptured it is unlikely to fail again (e.g. Calais et al. 2016). According to this interpretation, the locations of earthquakes migrate spatially and temporally, depending on the stress state. Both sets of these models predict locally elevated stress regions within the stable continental interior, and with only a few decades’ worth of quantitative seismic data, it is somewhat difficult to definitively disprove either. Let’s explore briefly how both of these models actually work.
Concentrated intraplate seismicity
The basic setup in this model set has a local heterogeneity in an otherwise “ambient” lithosphere.
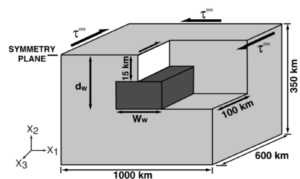
Figure: Numerical model suggested by Kenner and Segall (2000) to explain intraplate earthquakes. Light gray is elastic lithosphere, dark gray is the weak zone and white plane is the fault, under the far-field tectonic stresses.
This idea was demonstrated by Kenner and Segall (2000) using time-dependent numerical models employing a weak lower-crust embedded in an elastic lithosphere subject to far-field tectonic stresses. The basic physical process is that the weak zones cannot sustain enough stress over time and, therefore, transmits it to nearby faults. Over time, the stress exceeds the fault strength and an earthquake occurs. Fraction of stresses released during an earthquake accumulates back into the weak zone, thereby continuing the cycle of earthquakes. The location of the weak zone varies among different models, with most likely candidates being lower-crust or upper-mantle, but there is more variety in the origin of the weak zone. In addition to the weak zone, most models also require preexisting fault structures or fractured zones and stress-concentration mechanisms, e.g., tectonic loading, waster water injection, glacial rebound, or lithospheric foundering. We can usually find the links to the specific weakness and stress ingredients of the model in the tectonic and geological history of the region.
Migrating intraplate seismicity
The numerical models supporting this paradigm (e.g., Li et al., 2009) cover a much larger spatial domain and run for a longer time than the models above. These models are relatively straightforward: an elastic upper layer (upper crust) and a ductile lower layer (lower crust and upper mantle) under kinematic boundary conditions to achieve far-field stresses. The earthquakes are simulated randomly in the upper layer by reducing the strength of few elements. As stress increases through the model and surpasses the strength of the weak elements, the elements fail to generate earthquakes. The changes in the coseismic stress (during earthquakes) and the postseismic stress (viscous relaxation after earthquakes) of the failed elements determines if the stress in the nearby elements increases or decreases, thereby promoting or hindering earthquakes. The random seed of weak elements and continuous stress evolution lead to scattered seismicity within the entire model domain.
Modeling intraplate seismicity
The simplicity of the above geodynamic models might mislead us into thinking that we have understood the physics of intraplate earthquakes. Constructing such models require tedious assimilation of various geophysical data sets: gravity, seismic tomography, fault boundaries, heat flow, etc. Constrained with these observations, these models typically involve highly non-linear material properties (to appropriately represent the lithosphere), which can then self-consistently generate enough stress to cause the faults to fail and also match the expected spatial and temporal patterns of these earthquakes. If with all these complexities, our models give the expected behavior of earthquakes (implying that we accurately predicted the fault strength, lithospheric rheology, and buoyancy forces from the observations), there remains the question of if and how these zones of weakness were formed in the first place. The answer to this question requires detailed investigation into the region’s history by allowing the models to go backward in time, a topic we might have to revisit later.
Further reading: Kenner, Shelley J., and Paul Segall. ”A mechanical model for intraplate earthquakes: Application to the New Madrid seismic zone.” Science 289.5488 (2000): 2329-2332. Li, Qingsong, Mian Liu, and Seth Stein. "Spatiotemporal complexity of continental intraplate seismicity: insights from geodynamic modeling and implications for seismic hazard estimation." Bulletin of the Seismological Society of America 99.1 (2009): 52-60. Calais, Eric, et al. "A new paradigm for large earthquakes in stable continental plate interiors." Geophysical Research Letters 43.20 (2016): 10-621. Thomas, William A., and Christine A. Powell. "Necessary conditions for intraplate seismic zones in North America." Tectonics 36.12 (2017): 2903-2917.