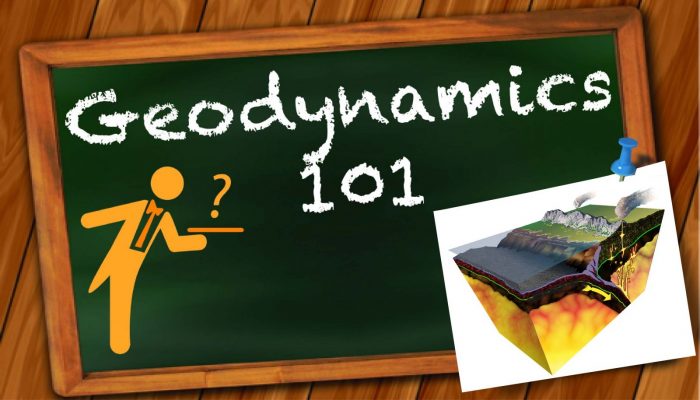
Subduction zones are ubiquitous features on Earth, and an integral part of plate tectonics. They are known to have a very important role in modulating climate on Earth, and are believed to have played an essential part in making the Earth’s surface habitable, a role that extends to present-day. This week, Antoniette Greta Grima writes about the ongoing debate on how subduction zones form and persist for millions of years, consuming oceanic lithosphere and transporting water and other volatiles to the Earth’s mantle.
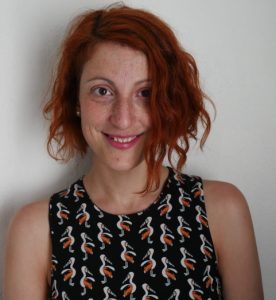
Antoniette Greta Grima. PhD Student at Dept. of Earth Sciences, University College London, UK.
Before we can start thinking about how subduction zones form, we need to be clear on what we mean by the term subduction zone. In the most generic sense, this term has been described by White et al. (1970) as “an abruptly descending or formerly descended elongate body of lithosphere, together with an existing envelope of plate deformation”. In simple words this defines subduction zones as places where pieces of the Earth’s lithosphere bend downwards into the Earth’s interior. This definition however, does not take into account the spatio-temporal aspect of subduction zone formation. It also, does not differentiate between temporary, episodic lithosphere ‘peeling’ or ‘drips’, thought to precede the modern-day ocean plate-tectonic regime (see van Hunen and Moyen, 2012; Crameri et al., 2018; Foley, 2018, and references therein) and the rigid self-sustaining subduction, which we see on the present-day Earth (Gurnis et al., 2004).
A self-sustaining subduction zone is one where the total buried, rigid slab length extends deep into the upper mantle and is accompanied at the surface by back-arc spreading (Gurnis et al., 2004). The latter is an important surface observable indicating that the slab has overcome the resistive forces impeding its subduction and is falling quasi-vertically through the mantle. Gurnis et al. (2004) go on to say that if one or the other of these defining criteria is missing then subduction is forced rather than self-sustaining. Forced or induced subduction (Stern, 2004; Leng and Gurnis, 2011; Stern and Gerya, 2017; Baes et al., 2018) is described by Gurnis et al. (2004) as a juvenile, early stage system, that cannot be described as a fully fledged subduction zone. These forced subduction zones are characterised by incipient margins, short trench-arc distance, narrow trenches and a volcanically inactive island arc and/or trench. Furthermore, although these juvenile systems might be seismically active they will lack a well defined Benioff Zone. Examples of forced subduction include the Puysegur-Fiordland subduction along the Macquarie Ridge Complex, the Mussau Trench on the eastern boundary of the Caroline plate and the Yap Trench south of the Marianas, amongst others. On the other hand, Cenozoic (<66 Ma) subductions, shown in figure 2, are by this definition self-sustaining and mature subduction zones. These subduction zones including the Izu-Bonin-Mariana, Tonga-Kermadec and Aleutians subduction zones, are characterised by their extensive and well defined trenches (see figure 2) (Gurnis et al., 2004). However, despite their common categorization subduction zones can originate through various mechanisms and from very different tectonic settings.
1. How are subduction zones formed?
We know from the geological record that the formation of subduction zones is an ongoing process, with nearly half of the present day active subduction zones initiating during the Cenozoic (<66 Ma) (see Gurnis et al., 2004; Dymkova and Gerya, 2013; Crameri et al., 2018, and references therein). However, it is less clear how subduction zones originate, nucleate and propagate to pristine oceanic basins.
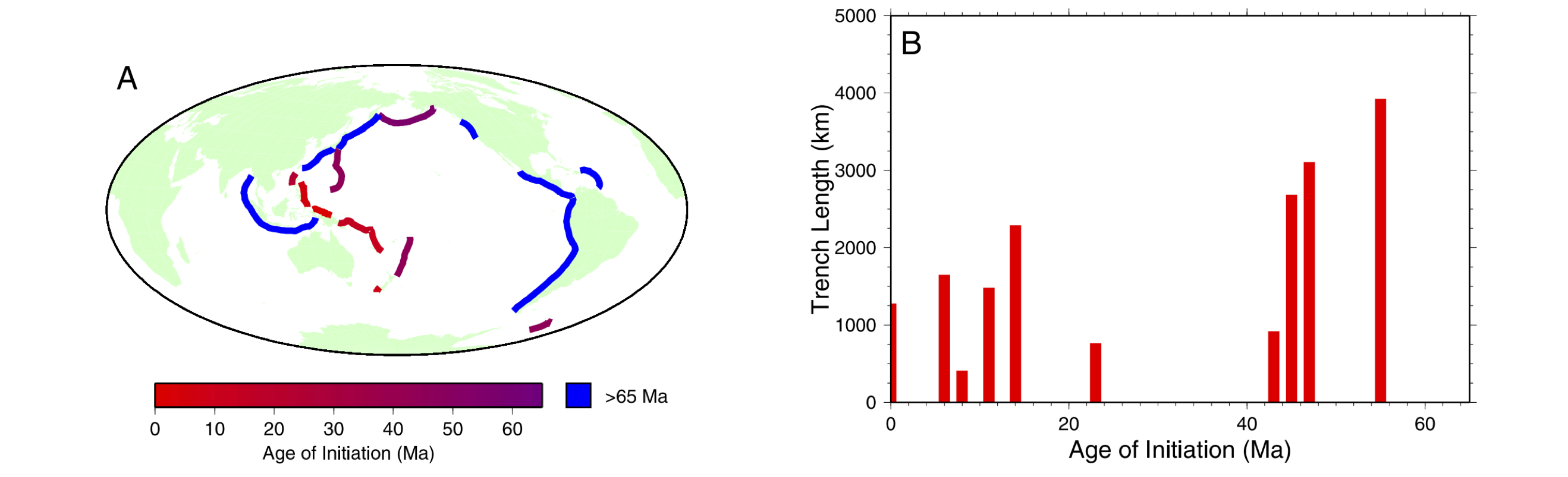
Figure 2: The oldest, still active subduction zones on Earth can be dated back to 66 million years ago. These are self- sustaining mature subduction zones with well defined trenches and trench lengths (modified from Gurnis et al., 2004).
Crameri et al. (2018, and references therein) list a number of mechanisms, some which are shown in figure 3, that may work together to weaken and break the lithosphere including:
- Meteorite impact
- Sediment loading
- Major episode of delamination
- Small scale convection in the sub-lithospheric mantle
- Interaction of thermo-chemical plume with the overlying lithosphere
- Plate bending via surface topographic variations
- Addition of water or melt to the lithosphere
- Pre-existing transform fault or oceanic plateau
- Shear heating
- Grain size reduction
Some of these mechanisms, particularly those listed at the beginning of the list are more appropriate to early Earth conditions while others, such as inherited weaknesses or fracture zones, transform faults and extinct spreading ridges are considered to be prime tectonic settings for subduction zone formation in the Cenozoic (<66 Ma) (Gurnis et al., 2004). As the oceanic lithosphere grows denser with age, it develops heterogeneity which facilitates its sinking into the mantle to form new subduction zones. However, it is important to keep in mind that without inherited, pre-existing weaknesses, it is extremely difficult to form subduction zones at passive margins. This is because as the oceanic lithosphere cools and becomes denser, it also becomes stronger and therefore harder to bend into the mantle that underlies it (Gurnis et al., 2004; Duarte et al., 2016). Gurnis et al. (2004) note that the formation of new subduction zones alters the force balance on the plate and suggest that the strength of the lithosphere during bending is potentially the largest resisting component in the development of new subduction zones. Once that resistance to bending is overcome, either through the negative buoyancy of the subducting plate and/or through the tectonic forces acting on it, a shear zone extending through the plate develops (Gurnis et al., 2004; Leng and Gurnis, 2011). This eventually leads to plate failure and subduction zone formation.
2. Where can new subduction zones form?
From our knowledge of the geological record, observations of on-going subduction, and numerical modelling (Baes et al., 2011; Leng and Gurnis, 2011; Baes et al., 2018; Beaussier et al., 2018) we think that subduction zone initiation primarily occurs through the following:
In an intra-oceanic setting through surface weakening processes
An intra-oceanic setting refers to a subduction zone forming right within the oceanic plate itself. Proposed weakening mechanisms include weakening of the lithosphere due to melt and/or hydration (e.g., Crameri et al., 2018; Foley, 2018, and references therein), localised lithospheric shear heating (Thielmann and Kaus, 2012) and density variations within oceanic plate due to age heterogeneities, where its older and denser portions flounder and sink (Duarte et al., 2016). Another mechanism proposed by Baes et al. (2018) suggests that intra-oceanic subduction can also be induced by mantle suction flow. These authors suggest that mantle suction flow stemming from either slab remnants and/or from slabs of active subduction zones can act on pre-existing zones of weakness, such as STEP (subduction-transfer edge propagate) faults to trigger a new subduction zone, thus facilitating spontaneous subduction initiation (e.g. figure 3) (Stern, 2004). The Sandwich and the Tonga-Kermadec subduction zones are often cited as prime examples of intra-oceanic subduction zone formation due to mantle suction forces (Baes et al., 2018). Ueda et al. (2008) and Gerya et al. (2015) also suggest that thermochemical plumes can break the lithosphere and initiate self-sustaining subduction, provided that the overlying lithosphere is weakened through the presence of volatiles and melt (e.g. figure 3). This mechanism can explain the Venusian corona and could have facilitated the initation of plate tectonics on Earth (Ueda et al., 2008; Gerya et al., 2015). Similarly Burov and Cloetingh (2010) suggest that in the absence of plate tectonics, mantle lithospheric interaction through plume-like instabilities, can induce spontaneous downwelling of both continental and oceanic lithosphere.
Through subduction infection or invasion
Subduction invasion/infection (Waldron et al., 2014; Duarte et al., 2016) occurs when subduction migrates from an older system into a pristine oceanic basin. Waldron et al. (2014) suggest that the closure of the Iapetus Ocean is due to the encroachment of old lithosphere into a young ocean. These authors suggests that subduction initiated at the boundary between old and new oceanic lithosphere and was introduced to the area through trench rollback. This process is thought be similar to the modern day Caribbean, Scotia and Gibraltar Arcs (Duarte et al., 2016). This suggests that the older subductions of the Pacific are invading the younger Atlantic basin, which might potentially lead to collision, orogeny and closure of the Atlantic ocean (Duarte et al., 2016).
Following a subduction polarity reversal
Subduction polarity reversal describes a process where the trench jumps from the subducting plate to the overriding one, flipping its polarity in the process (see figure 3). This can result from the arrival at the trench of continental lithosphere (McKenzie, 1969) or young positively buoyant lithosphere (Crameri and Tackley, 2015). Subduction polarity reversal is often invoked to explain and justify the two juxtaposed Wadati-Benioff zones and their opposite polarities, in the Solomon Island Region (Cooper and Taylor, 1985). Indications of a polarity reversal are also exhibited below the Alpine and Apennine Belts (Vignaroli et al., 2008). Furthermore, Crameri and Tackley (2014) also suggest that the continental connection between South America and the Antarctic peninsula has been severed through a subduction polarity reversal, resulting in the lateral detachment of the South Sandwich subduction zone.
Subduction initiation at ancient/ inherited zones of lithospheric weakness
Subduction zones can also initiate at ancient, inherited zones of weakness such as old fracture zones, transform faults, extinct subduction boundaries and extinct spreading ridges (Gurnis et al., 2004). Gurnis et al. (2004) suggest that the Izu-Bonin-Mariana subduction zone initiated at a fracture zone, while the Tonga-Kermadec subduction initiated at an extinct subduction boundary. The same study also proposes that the incipient Puysegur-Fiordland subduction zone nucleated at an extinct spreading centre.
In conclusion, we can say that subduction zone formation is a complex and multi layered process that can stem from a variety of tectonic settings. However, it is clear that our planet’s current convection style, mode of surface recycling and its ability to sustain life are interlinked with subduction zone formation. Therefore, to understand better how subduction zones form is to better understand what makes the Earth the planet it is today.
References: Baes, M., Govers, R., and Wortel, R. (2011). Subduction initiation along the inherited weakness zone at the edge of a slab: Insights from numerical models. Geophysical Journal International, 184(3):991–1008. Baes, M., Sobolev, S. V., and Quinteros, J. (2018). Subduction initiation in mid-ocean induced by mantle suction flow. Geophysical Journal International, 215(3):1515–1522. Beaussier, S. J., Gerya, T. V., and Burg, J.-p. (2018). 3D numerical modelling of the Wilson cycle: structural inheritance of alternating subduction polarity. Fifty years of the Wilson Cycle concept in plate tectonics, page First published online. Burov, E. and Cloetingh, S. (2010). Plume-like upper mantle instabilities drive subduction initiation. Geophys. Res. Lett., 37(3). Cooper, P. and Taylor, B. (1985). Polarity reversal in the Solomon Island Arc. Nature, 313(6003):47–48. Crameri, F., Conrad, C. P., Mont ́esi, L., and Lithgow-Bertelloni, C. R. (2018). The dynamic life of an oceanic plate. Tectonophysics. Crameri, F. and Tackley, P. J. (2014). Spontaneous development of arcuate single-sided subduction in global 3-D mantle convection models with a free surface. Journal of Geophysical Research: Solid Earth, 119(7):5921–5942. Crameri, F. and Tackley, P. J. (2015). Parameters controlling dynamically self-consistent plate tectonics and single-sided subduction in global models of mantle convection. Journal of Geophysical Research: Solid Earth, 3(55):1–27. Duarte, J. C., Schellart, W. P., and Rosas, F. M. (2016). The future of Earth’s oceans: Consequences of subduction initiation in the Atlantic and implications for supercontinent formation. Geological Magazine, 155(1):45–58. Dymkova, D. and Gerya, T. (2013). Porous fluid flow enables oceanic subduction initiation on Earth. Geophysical Research Letters, 40(21):5671–5676. Foley, B. J. (2018). The dependence of planetary tectonics on mantle thermal state : applications to early Earth evolution. 376. Gerya, T. V., Stern, R. J., Baes, M., Sobolev, S. V., and Whattam, S. A. (2015). Plate tectonics on the Earth triggered by plume-induced subduction initiation. Nature, 527(7577):221–225. Gurnis, M., Hall, C., and Lavier, L. (2004). Evolving force balance during incipient subduction. Geochemistry, Geophysics, Geosystems, 5(7). Leng, W. and Gurnis, M. (2011). Dynamics of subduction initiation with different evolutionary pathways. Geochemistry, Geophysics, Geosystems, 12(12). McKenzie, D. P. (1969). Speculations on the Consequences and Causes of Plate Motions. Geophys. J. R. Astron. Soc., 18(1):1–32. Stern, R. J. (2004). Subduction initiation: spontaneous and induced. Earth and Planetary Science Letters, 226(3-4):275–292. Stern, R. J. and Gerya, T. (2017). Subduction initiation in nature and models: A review. Tectonophysics. Thielmann, M. and Kaus, B. J. (2012). Shear heating induced lithospheric-scale localization: Does it result in subduction? Earth Planet. Sci. Lett., 359-360:1–13. Ueda, K., Gerya, T., and Sobolev, S. V. (2008). Subduction initiation by thermal-chemical plumes: Numerical studies. Phys. Earth Planet. Inter., 171(1-4):296–312. van Hunen, J. and Moyen, J.-F. (2012). Archean Subduction: Fact or Fiction? Annual Review of Earth and Planetary Sciences, 40(1):195–219. Vignaroli, G., Faccenna, C., Jolivet, L., Piromallo, C., and Rossetti, F. (2008). Subduction polarity reversal at the junction between the Western Alps and the Northern Apennines, Italy. Tectonophysics, 450(1-4):34–50. Waldron, J. W., Schofield, D. I., Brendan Murphy, J., and Thomas, C. W. (2014). How was the iapetus ocean infected with subduction? Geology, 42(12):1095–1098. White, D. A., Roeder, D. H., Nelson, T. H., and Crowell, J. C. (1970). Subduction. Geological Society of America Bulletin, 81(October):3431–3432. W.K, H. and E.H., C. (2003). Earth’s Dynamic Systems. Prentice Hall; 10 edition.