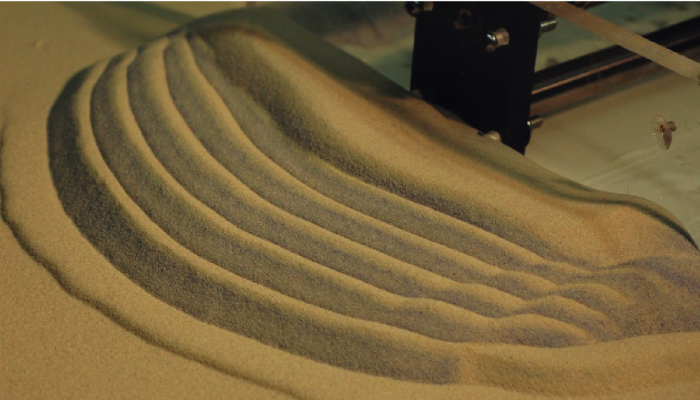
In geodynamics we study the dynamics of the Earth (and other planets). We ground our studies in as much data as possible, however we are constrained by the fact that pretty much all direct information we can collect from the interior of the Earth only shows its present-day state. The surface rock record gives us a glimpse into the past dynamics and evolution of our planet, but this record gets sparser as we go back in time. This is why it is common to use modelling in geodynamics to fill this gap of knowledge. There are different types of modelling, and this week João Duarte writes about the importance of analogue modelling.
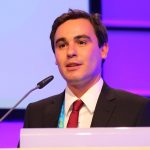
João Duarte. Researcher at Instituto Dom Luiz and Invited Professor at the Geology Department, Faculty of Sciences of the University of Lisbon. Adjunct Researcher at Monash University.
The first time I went to EGU, in 2004, I presented a poster with some new marine geology data and a few sets of analogue models. I was doing accretionary wedges in sandboxes. At the time, I was in the third year of my bachelor’s degree and I was completely overwhelmed by the magnitude of the conference. It was incredible to see the faces of all those scientists that I used to read articles from. But one thing impressed me the most. Earth Sciences were consolidating as a modern, theoretical based science. The efforts in trying to develop an integrated dynamic theory of plate tectonics as part of mantle convection were obvious. The new emergent numerical models looked incredible, with all those colours, complex rheologies and stunning visualization that allowed us to “see” stresses, temperature gradients and non-linear viscosities. I was amazed.
Analogue modelling was at a relative peak in 2004, however it was also anticipated by some that it would quickly disappear (and indeed several analogue labs have closed since). It was with this mindset, that I later did the experiments for my PhD, which I finished in 2012 (Duarte et al., 2011). But I was fortunate. My supervisors, Filipe Rosas and Pedro Terrinha, took me to state-of-art labs, namely Toronto and Montpellier (lead at the time by Sandy Cruden and Jacques Malavieille, respectively), and I started to develop a passion for this kind of models. When I moved to Monash for a post-doc position, in 2011, this turned out to be a great advantage. There, modelers such as Wouter Schellart, Louis Moresi, Fabio Capitanio, David Boutelier and Sandy Cruden (yes, I met Sandy again at Monash) were using analogue models to benchmark numerical models. Why? Because many times, even though numerical models produce spectacular results, they might not be physically consistent. And there is only one way to get rid of this, which is to make sure that whatever numerical code we are using can reproduce simple experiments that we can run in a lab. The classical example is the sinking of a negatively buoyant sphere in a viscous medium.
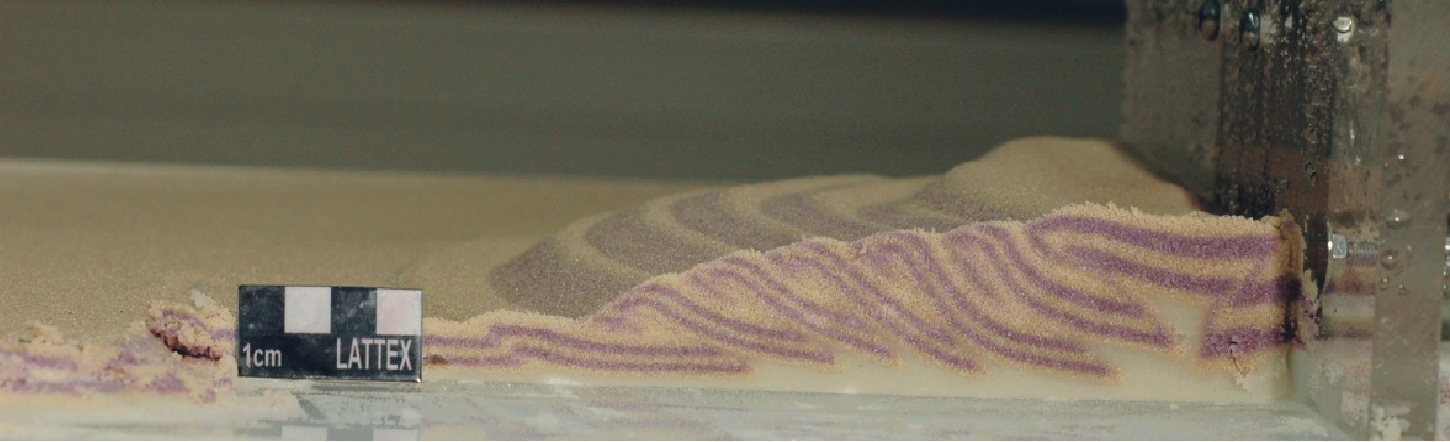
Sandbox analogue model of an accretionary wedge. Part of the same experiment as shown in the header figure. Here, a sliced section cut after wetting, is shown. University of Lisbon, 2009. Experiments published in Duarte et al. (2011).
That was what we were doing at Monash. I worked with Wouter Schellart in the development of subduction experiments with an overriding plate, which were advancing step by step in both analogue and numerical schemes (see e.g., Duarte et al., 2013 and Chen et al., 2015, 2016 for the analogue models, and Schellart and Moresi, 2013 for numerical equivalents). The tricky bit was, we wanted to have self-consistent dynamic experiments in which we were ascribing the forces (negative buoyancy of the slab, the viscosity of the upper mantle, etc) and let the kinematics (i.e. the velocities) to be an emergent phenomenon. So, no lateral push or active kinematic boundaries were applied to the plates. This is because we now recognize that in general, it is the slab pull at subduction zones that majorly drives the plates and not the other way around. Therefore, if we want to investigate the fundamental physics and dynamics of subduction zones we need to use self-consistent models (both analogue and numerical). In order to carry out these models, we had to develop a new rheology for the subduction interface, which is a complex problem, both in the analogue and the numerical approaches (Duarte et al. 2013, 2014, 2015). But this is another very long story that would lead to a publication by itself.
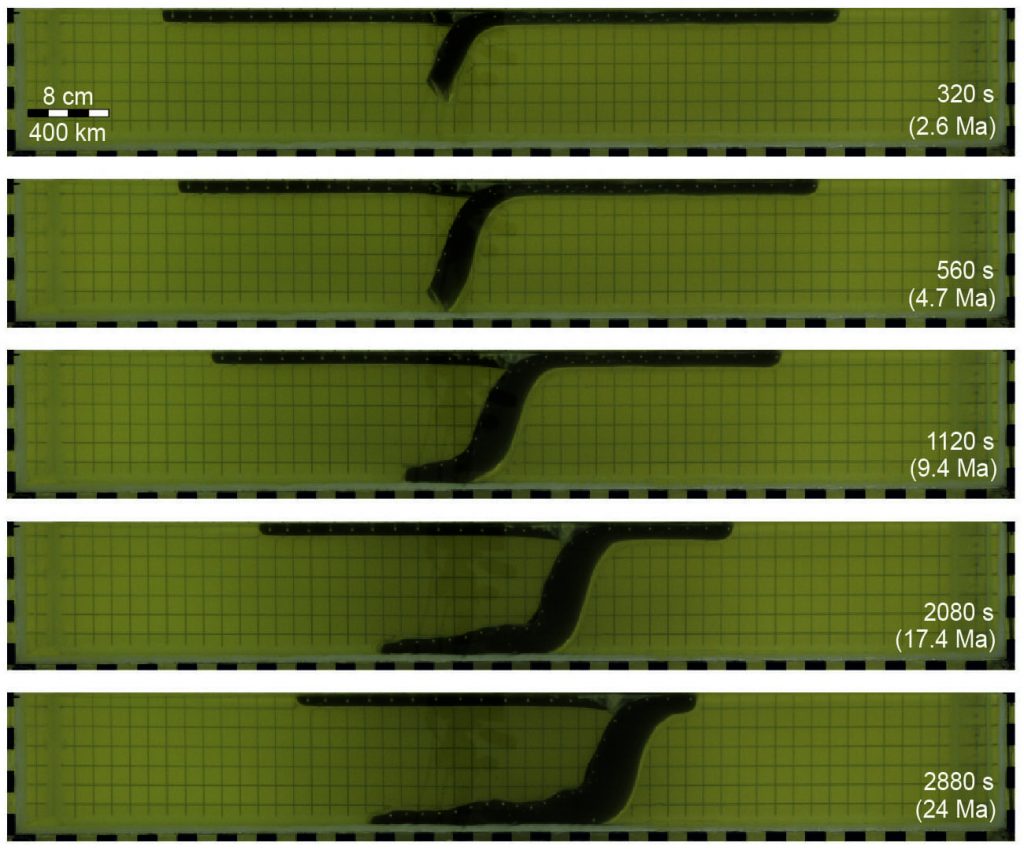
Analogue models of subduction with an overriding plate and an interplate rheology. Monash University, 2012. Adapted from Duarte et al. (2013)
But what is analogue modelling all about? Basically, analogue models are scaled models that we can develop in the laboratory using analogue materials (such as sand), and that at the scale that we are doing our models have similar physical properties to those of natural materials (such as brittle rocks). But, as it is widely known, under certain circumstances (at large time and space scales), rocks behave like fluids, and for that we use analogue fluids, such as silicone putties, glucose and honey. We can also use fluids to simulate the interaction between subduction zones and mantle plumes in a fluid reservoir (see below figures and links to videos of scaled experiments using three different fluids to study slab-plume interaction; Meriaux et al., 2015a, 2015b, 2016). These are generally called geodynamic analogue models.
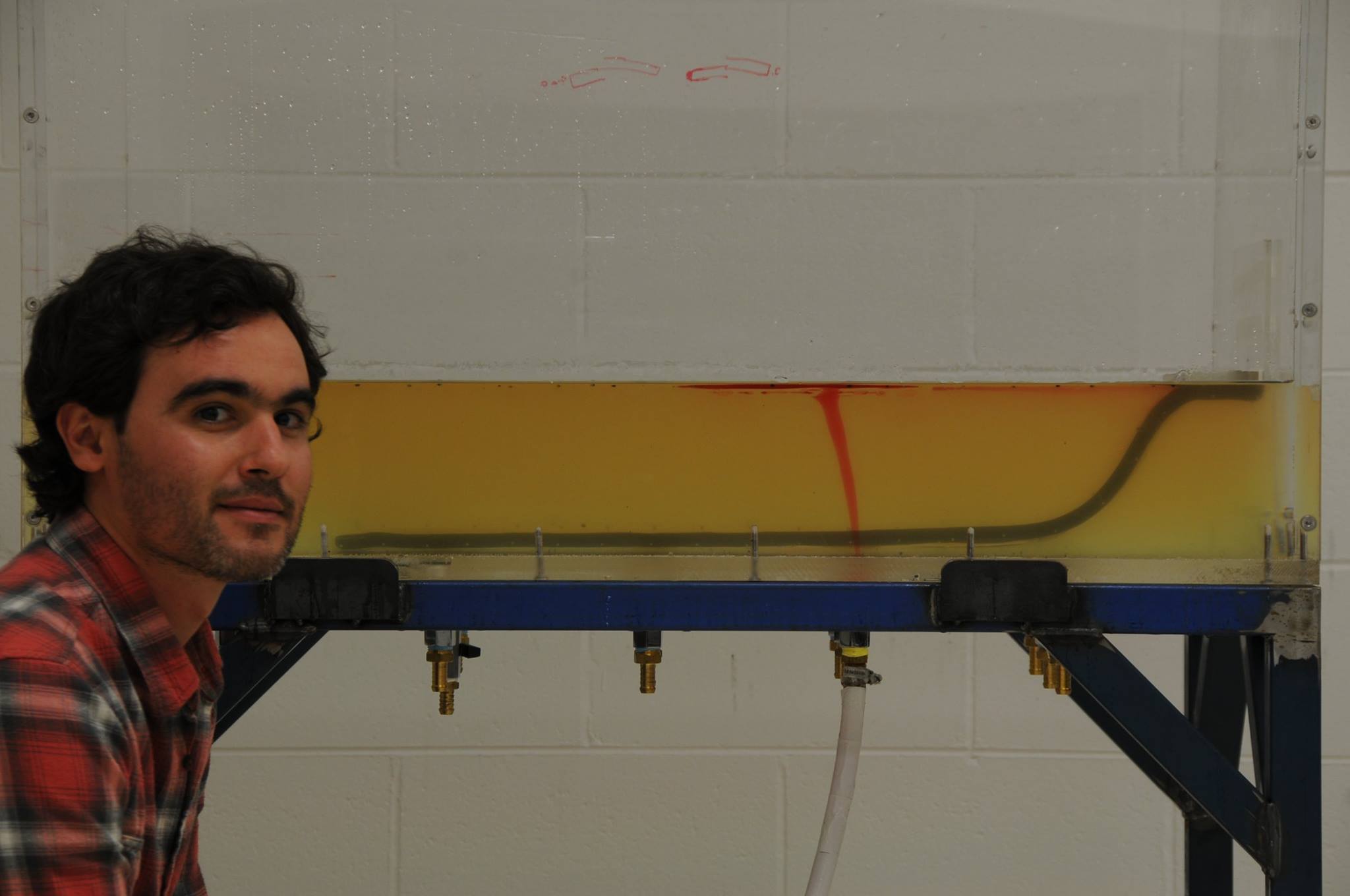
End of a slab-plume experiment in the upper mantle (see below). The tank is partially filled with glucose. The slab (laying at the analogue 660 discontinuity) is made of silicone mixed with iron powder. The plume is made of a water solution of glucose dyed with a red colorant. And that’s me on the left. Monash University, 2014.
I usually consider two main branches of analogue models. The first, which is the one mostly used by geologists, was started by Sir James Hall (1761 – 1832), that squeezed layers of clay to reproduce the patterns of folded rocks that he had observed in nature. This method was later improved by King Hubbert (1937), who laid the ground for the development of the field by developing a theory of scaling of analogue models applied to geological processes.
The other branch is probably as old as humans. It began when we started to manipulate objects and using them to understand basic empirical laws, such as the one that objects always fall. When Galileo was using small spheres in inclined surfaces to extract the physical laws that describe the movement of bodies, from rocks to planets, he was in a certain way using analogue models. He understood that many laws are scale invariant. Still today, these techniques are widely used by physicist and engineers when understanding for example the aerodynamics of airplanes, the stability of bridges, the dynamics of rivers or the resistance of dams. They use scaled models that reproduce at suitable laboratory scales the objects and processes that they are investigating.
What we did at Monash, was a mixture of both approaches. Though, we were less interested in exactly reproducing nature from a purely geometric and kinematic point of view, but we were more interested in understanding the physics of the object we were investigating: subduction zones. Therefore, we had to guarantee that we were using the correct dynamical approach in order to be able to extract generic physical empirical laws, hoping that these laws would provide us some insight on the dynamics of natural subduction zones. These empirical laws could readily be incorporated in numerical models, which would then help exploring more efficiently the space of the controlling parameters in the system.
I want to finish with a question that I believe concerns all of us: are there still advantages in using analogue models? Yes, I believe so! One of the most important advantages is that analogue models are always three-dimensional and high-resolution. Furthermore, they allow a good tracking of the strain and to understand how it occurs in discontinuous mediums, for example when investigating the localization of deformation or the propagation of cracks. Numerical schemes still struggle with these problems. It is very difficult to have an efficient code that can deal simultaneously with very high resolution and large-scale three-dimensional problems, as it is required to investigate the process of subduction. Nevertheless, numerical models are of great help when it comes to track stresses, and model complex rheologies and temperature gradients. To sum up: nowadays, we recognize that certain problems can only be tackled using self-consistent dynamic models that model the whole system in three-dimensions, capturing different scales. For this, the combination of analogue and numerical models is still one of the most powerful tools we have. An interesting example of a field in which a combined approach is being used is the fascinating investigations on the seismic cycle (for example, see here).
Links to videos: VIDEO 1: https://www.youtube.com/watch?v=U1TXC2XPbFA&feature=youtu.be (Subduction with an overriding plate and an interplate rheology. Duarte et al., 2013) VIDEO 2: https://www.youtube.com/watch?v=n5P2TzS6h_0&feature=youtu.be (Slab-plume interaction at mantle scale. Side-view of the experiment on the top, and top-view of the experiment on the bottom. Meriaux et al., 2016)
References: Chen, Z., Schellart, W.P., Strak, V., Duarte, J.C., 2016. Does subduction-induced mantle flow drive backarc extension? Earth and Planetary Science Letters 441, 200-210. https://doi.org/10.1016/j.epsl.2016.02.027 Chen, Z., Schellart, W.P., Duarte, J.C., 2015. Overriding plate deformation and variability of forearc deformation during subduction: Insight from geodynamic models and application to the Calabria subduction zone. Geochemistry, Geophysics, Geosystems 16, 3697–3715. DOI: 10.1002/2015GC005958 Duarte, J.C., Schellart, W.P., Cruden, A.R., 2015. How weak is the subduction zone interface? Geophysical Research Letters 41, 1-10. DOI: 10.1002/2014GL062876 Duarte, J.C., Schellart, W.P., Cruden, A.R., 2014. Rheology of petrolatum – paraffin oil mixtures: applications to analogue modelling of geological processes. Journal of Structural Geology 63, 1-11. https://doi.org/10.1016/j.jsg.2014.02.004 Duarte, J.C., Schellart, W.P., Cruden, A.R., 2013. Three-dimensional dynamic laboratory models of subduction with an overriding plate and variable interplate rheology. Geophysical Journal International 195, 47-66. https://doi.org/10.1093/gji/ggt257 Duarte, J.C., F.M. Rosas P., Terrinha, M-A Gutscher, J. Malavieille, Sónia Silva, L. Matias, 2011. Thrust–wrench interference tectonics in the Gulf of Cadiz (Africa–Iberia plate boundary in the North-East Atlantic): Insights from analog models. Marine Geology 289, 135–149. https://doi.org/10.1016/j.margeo.2011.09.014 Hubbert, M.K., 1937. Theory of scale models as applied to the study of geologic structures. GSA Bulletin 48, 1459-1520. https://doi.org/10.1130/GSAB-48-1459 Meriaux, C., Meriaux, A-S., Schellart, W.P., Duarte, J.C., Duarte, S.S., Chen, Z., 2016. Mantle plumes in the vicinity of subduction zones. Earth and Planetary Science Letters 454, 166-177. https://doi.org/10.1016/j.epsl.2016.09.001 Mériaux, C.A., Duarte, J.C., Schellart, W.P., Mériaux, A-S., 2015. A two-way interaction between the Hainan plume and the Manila subduction zone. Geophysical Research Letters 42, 5796–5802. DOI: 10.1002/2015GL064313 Meriaux, C.A., Duarte, J.C., Duarte, S., Chen, Z., Rosas, F.M., Mata, J., Schellart, W.P., and Terrinha, P. 2015. Capture of the Canary mantle plume material by the Gibraltar arc mantle wedge during slab rollback. Geophysical Journal International 201, 1717-1721. https://doi.org/10.1093/gji/ggv120 Schellart, W.P., Moresi, L., 2013. A new driving mechanism for backarc extension and backarc shortening through slab sinking induced toroidal and poloidal mantle flow: Results from dynamic subduction models with an overriding plate. Journal of Geophysical Research: Solid Earth 118, 3221-3248. https://doi.org/10.1002/jgrb.50173