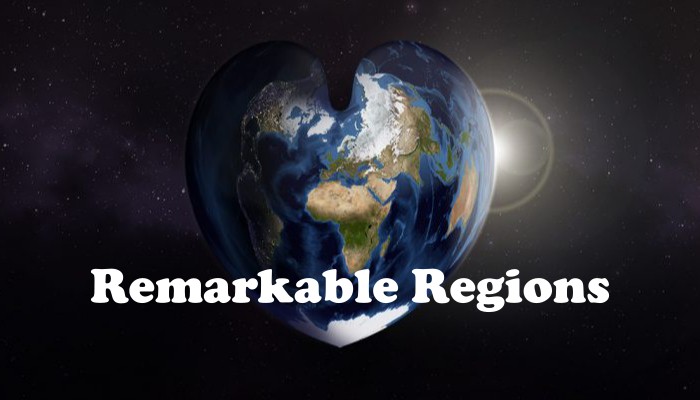
Every 8 weeks we turn our attention to a Remarkable Region that deserves a spot in the scientific limelight. This week we zoom in on a particular part of the eastern Tethys as Adina Pusok discusses the India-Asia collision zone. She is a postdoctoral researcher at the Institute of Geophysics and Planetary Physics, Scripps Institution of Oceanography, UCSD, US.
Without doubt, one of the most striking features of plate tectonics and lithospheric deformation on Earth is the India-Asia collision zone, largely comprised of the Himalayan and Karakoram mountain belts and the Tibetan plateau. What makes this collision zone so remarkable? For one, Tibet is the largest, highest and flattest plateau on Earth with an average elevation exceeding 5 km, and it includes over 80% of the world’s land surface higher than 4 km. Then, the bordering Himalayas and the Karakoram Mountains include the only peaks on Earth reaching more than 8 km above sea level.
It makes one wonder, how can such a mountain belt and high plateau form? Most of the major mountain belts and orogenic plateaus on Earth are found within the overlying plate of subduction and/or collision zones (e.g. the Alps, the Andes, the American Cordilleras etc.). When an ocean closes and two continental plates meet at a destructive (subduction) boundary, the continents themselves collide. Such collisions result in intense deformation at the edges of the colliding plates. Neither continent can be subducted into the mantle due to the buoyancy of continental crust, so the forces that drive the plate movement prior to collision are brought to act directly on the continental lithosphere itself. At this stage, further convergence of the plates must be taken up by deforming one or both of the plates of continental lithosphere over hundreds of kilometres [Figure 1]. Mountain belts can form under these circumstances.
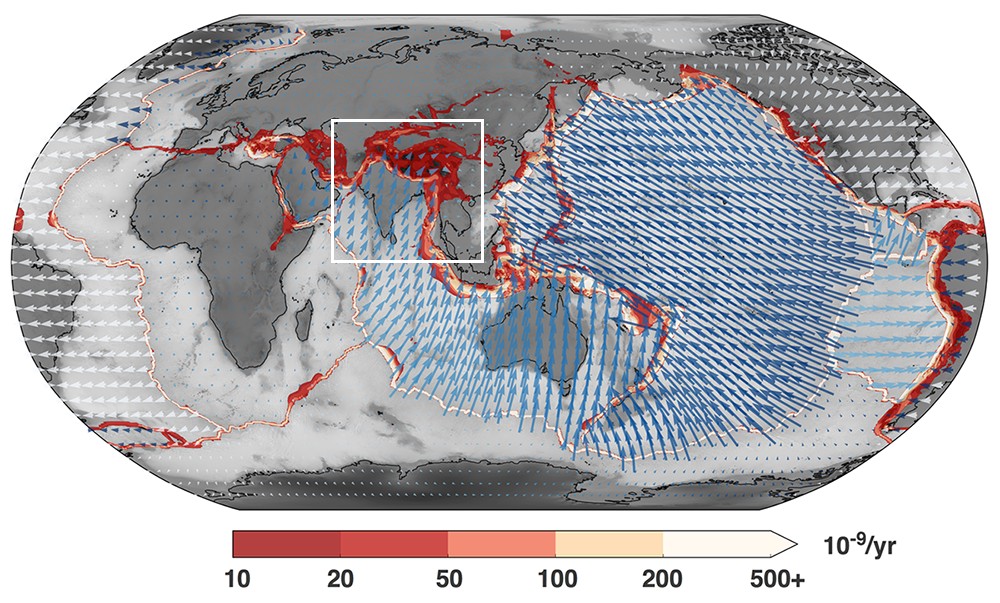
Figure 1 Global map of surface velocities and the second invariant of strain rate (from Moresi [2015]). The surface velocities show the location and extent of plates, and the strain rate map highlights the fact that most of the deformation is concentrated at plate boundaries (high strain rates), while the continental interiors have little or no deformation (low strain rates). In some places, deformation occurs over broader regions, especially following mountain belts. These boundaries are called diffuse plate boundaries. The white rectangle roughly indicates the extent of the India-Asia collision zone.
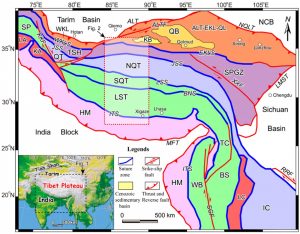
Figure 2 Simplified tectonic map of Tibet and surrounding region showing approximate boundaries of the major terranes, suture zones, and strike-slip faults (from Ninomiya and Bihong [2016]). Blocks and terranes: ALT-EKL-QL: Altyn Tagh–East Kunlun–Qilian terrane; BS: Baoshan terrane; HM: Himalayan terrane; IC: Indo-China block; KA: Kohistan Arc terrane; LA: Ladakh arc terrane; LC: Lincang–Sukhothai–Chanthaburi Arc terrane; LST: Lhasa terrane; NCB: North China Block; NQT: North Qiangtang terrane; QT: Qiangtang terrane; SP: South Pamir terrane; SPGZ: Songpan-Ganze terrane; SQT: South Qiangtang terrane; TC: Tengchong terrane; TSH: Tianshuihai terrane; WB: West Burma terrane; WKL: West Kunlun terrane. Suture zones: BNS: Bangong-Nujiang Suture; EKLS: East Kunlun Suture; ITS: Indus-Tsangbo Suture; JSS: Jinsha Suture; LSS: Longmu Tso–Shuanghu–Menglian–Inthanon Suture; WKLS: West Kunlun Suture. Basins: QB: Qaidam Basin; KB: Kumkol Basin. Faults: ALT: Altyn Tagh Thrust; ALTF: Altyn Tagh Fault; KKF: Karakorum Fault; LMST: Longmen Shan Thrust; MFT: Main Frontal Thrust; NQLT: North Qilian Thrust; RRF: Red River Fault; SGF: Sagaing Fault; XXF: Xianshui River–Xiaojiang Fault.
Interestingly, the India-Asia collision orogen is not just the youngest and most spectacular active continent collision belt, it is also the most studied research area on Earth. Studies on this region span a wide range of topics and methods for over more than 100 years. I am not sure if it is the fascination with the highest mountain on Earth (Mt. Everest was actually climbed for the first time as late as 1953 by Tenzing Norgay and Edmund Hillary), similar to our fascination for exploring the Moon, Mars and the other planets in our Solar System nowadays, or the hope that studying the youngest orogeny will help us decipher the older ones (soon to realize different mountain belts evolve differently).
To understand the magnitude of the work done in the past 100 years, a simple search of the keywords “India Asia collision” on Google Scholar yielded ~90k results, and a more focused geosciences search on Web of Science (where I filtered the results to those from geophysics, geochemistry, geology, geosciences multidisciplinary only) yielded >1600 results for the same keywords (other keywords: “Himalaya” > 5600 results, “Tibet” > 6500 results, “India Asia” > 2200 results). These numbers can be intimidating to a new student taking on the topic, but it is a topic worth studying and I’ll explain why below.
From a general perspective, it is important to study the India-Asia collision zone due to the interaction between tectonics and climate and the formation of the Indian monsoon [Molnar et al., 1993], but also because it is a highly populated area (>200 million people in the Hindu Kush Himalaya region) regularly shaken by natural phenomena, such as earthquakes, floods or landslides. For example, the last large earthquake in Nepal, the Gorkha earthquake (Mw 7.8) in April 2015 caused more than 9000 deaths.
From a geophysics point of view, understanding mountain-building processes and the driving forces of plate tectonics has been one of the long-term goals of solid Earth sciences community. The India-Asia collision zone is one of the best examples in which subduction, continental collision and mountain building can be studied in a global plate tectonics perspective. Prior to plate tectonics theory, Argand [1924] and Holmes [1965] thought that the Himalayan Mountains and Tibetan Plateau had been raised due to the northern edge of the Indian craton underthrusting the entire region, causing shortening and thickening of the crust to ∼80 km. This perspective remains widely accepted, but recent ideas suggest that other processes are equally important (more below).
Today, the challenge lies in refining our understanding of the dynamics of India-Asia collision by elucidating the connections between the wealth of observations available and the underlying processes occurring at depth. Decades of study have produced data sets across various disciplines, including: active tectonics, Cenozoic geology, seismicity, global positioning system (GPS) measurements, seismic profiles, tomography, gravity anomalies, mantle-crustal anisotropy, paleomagnetism, geochemistry or magnetotelluric studies. Of these, the GPS data stands out as it clearly shows the distributed deformation across the entire collision zone and suggests that this is a highly dynamic area [Figure 3].
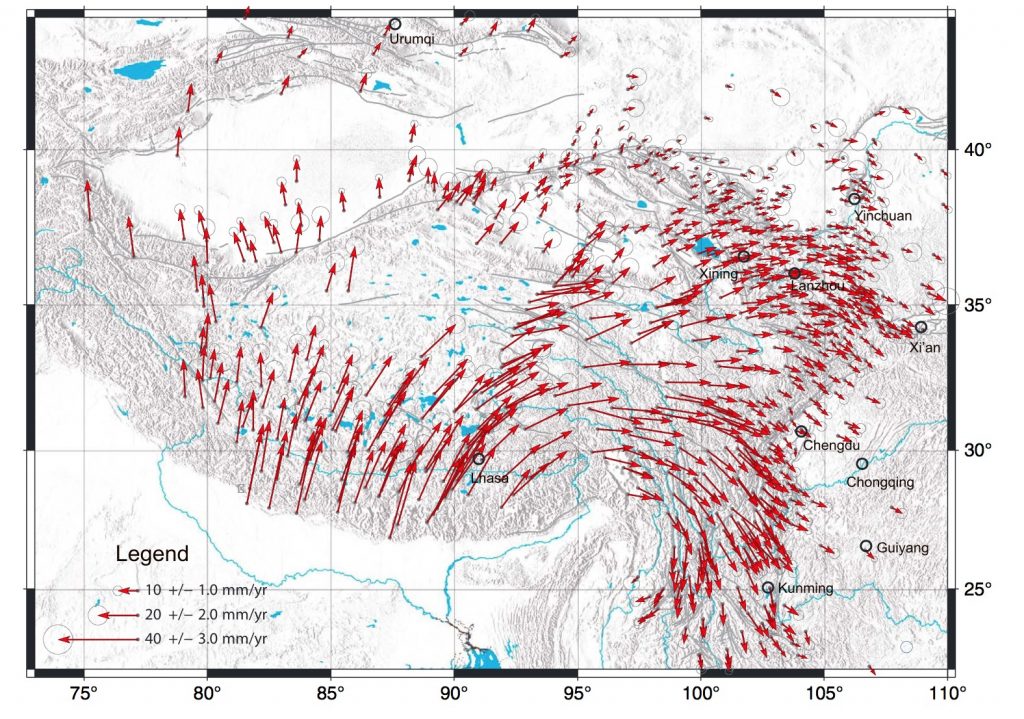
Figure 3 Horizontal GPS velocities of crustal motion around the Tibetan Plateau relative to stable Eurasia from Liang et al. [2013].
-
Figure 4 Schematic cartoons of tectonic models proposed to explain the thickening and uplift of the Himalayas and the Tibetan Plateau. (Source: personal institutional web page of A. Ozacar).
Wholescale underthrusting of the Indian plate below the Asian continent [e.g. Argand, 1924].
- The thin-sheet model or distributed homogeneous shortening [e.g. England and McKenzie, 1982].
- Homogeneous thickening of a weak, hot Asian crust, involving a large amount of magmatism [e.g. Dewey and Burke, 1973].
- Slip-line field model to account for the brittle deformation in and around the Tibetan Plateau and to explain extrusion of SE Tibet away from Indian continent [e.g. Molnar and Tapponnier, 1975]. The same group also proposes a time-dependent model for the growth of Tibetan plateau [e.g. Tapponnier et al., 2001], in which successive intracontinental subduction zones maintain the stepwise growth and rise of the plateau.
- Lower crustal flow models for the exhumation of the Himalayan units and lateral spreading of the Tibetan plateau [e.g. Royden et al., 1997, Beaumont et al., 2001].
- Delamination or convective removal of the lithospheric mantle that induced isostatic movement, lifting the Tibetan Plateau [e.g. Molnar, 1988].
These models were applied either to the Tibetan Plateau or the Himalayan mountain belt and were able to explain the formation of specific tectonic and geological features. However, there is no conclusive answer on which of the hypotheses works best for the entire orogen, and instead, more questions arise:
- Which forces are at work during continental collision and mountain building?
- What is the deformation history and evolution of this plate boundary?
- How was the subduction accommodated in the Neo-Tethys?
- How does subduction evolve during continental collision?
- What drives the present-day fast convergence (~4-5 cm/yr) between India and Eurasia?
- Which forces propagated India northwards between 70-50 million years at anomalously high speeds (up to 16 – 20cm/yr)?
- How can you form such large elevations over such extended areas?
- What is the effect of surface processes on uplift?
- What is the structure at depth beneath the Himalayas and Tibetan plateau?
- How do the Indian and Eurasia plates deform during collision?
- How is the deformation accommodated during continental collision?
- How do mountain belts form and why not all mountain belts look the same?
- How did the crust beneath Himalaya and Tibet reach double-crustal thickness (normal continental crust is 35-40 km thick, whereas the crust beneath the Himalaya and Tibet is 70-100 km thick)?
- Which mechanisms help sustain the high topographic amplitudes?
- Why should an area as broad as the Tibetan Plateau be uplifted so high compared to other mountain belts following collision?
- Did the Tibetan Plateau and Himalayan mountain belt rise continuously or diachronously?
- Which the proposed models [Figure 4] can be applied, and where?
- How do lithospheric heterogeneities and rheology affect the deformation pattern?
- What is the degree of mechanical coupling between the crust and deeper lithosphere? Is it the “jelly sandwich” model (e.g., Burov and Watts [2006]) or the “creme-brulee” model (e.g., Jackson [2002], see earlier blog post)?
- Why do the Himalayas have a convex curvature?
- What about the high deformation of the prominent Himalayan syntaxes (the inflection points of the Himalayan belt): Nanga Parbat in the west and Namche Barwa in the east?
- What is the effect of the India-Asia collision on climate? Do the Himalayas affect the Indian monsoon or is it the other way around? A chicken-and-egg question.
Seriously, can I even stop asking questions? The question that fascinated me the most during my graduate studies was “Why is the Himalayan-Tibet region so high and broad compared to other mountain belts?”. If we tune our models to Earth parameters, can we build such large elevations in computer simulations? Which factors and forces are at play? Using 3-D numerical models to address this question [Pusok and Kaus, 2015], we were able to obtain distinct topographic modes (different types of mountain belts) [Figure 5] and to show that building topography is an interplay between providing the energy to the system and the ability of that system to store it over longer periods of time. We also suggest that the reason why Himalaya-Tibet is different from the Alps, for example, is because the shape and elevation of mountain ranges can vary depending on the boundary conditions (plate driving forces that control convergence velocity and lithospheric heterogeneities such as the Tarim Basin) and internal factors (rheology), but also on the evolution stage they are in.
To sum up, it is clear that many of the above questions remain unanswered. But I think this is good news, meaning that in the future, exciting new results will shape our understanding of this remarkable region.
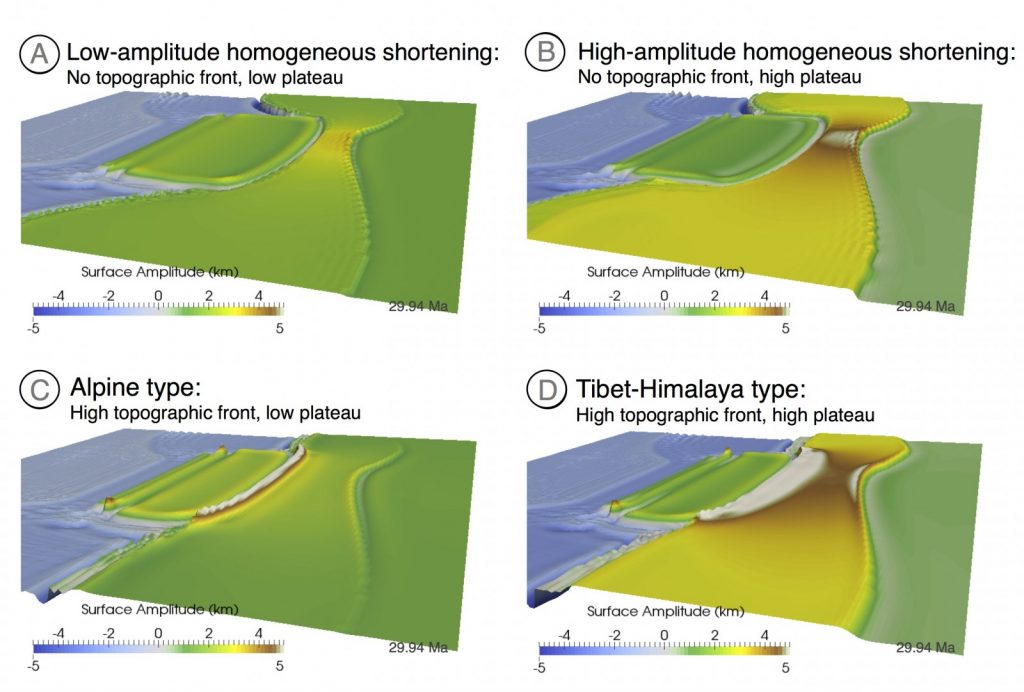
Figure 5 3-D Simulation results showing different modes of surface expressions in continental collision models. Modified from Pusok and Kaus [2015].
References: Argand, E. (1924). La tectonique de l’Asie. Proc. 13th Int. Geol. Cong., 7:171–372. Beaumont, C., Jamieson, R. A., Nguyen, M. H., and Lee, B. (2001). Himalayan Tectonics Explained by Extrusion of a Low-Viscosity Crustal Channel Coupled to Focused Surface Denudation. Nature, 414:738–742. Burov, E. B. and Watts, A. B. (2006). The long-term strength of continental lithosphere: “jelly sandwich” or “crème brûlée”? GSA Today, 16(1):4. Dewey, J. F. and Burke, K. (1973). Tibetan, Variscan, and Precambrian Basement Reactivation: Products of Continental Collision. The Journal of Geology, 81(6):683–692. England, P. and McKenzie, D. (1982). A Thin Viscous Sheet Model for Continental Deformation. Geophys. J. R. astr. Soc., 70:295–321. Holmes, A. (1965). Principles of Physical Geology. The Ronald Press Company, New York, second edition. Jackson, J. (2002). Strength of the Continental Lithosphere: Time to Abandon the Jelly Sandwich? GSA Today, 4–9. Liang, S., Gan, W., Shen, C., Xiao, G., Liu, J., Chen, W., Ding, X., and Zhou, D. (2013). Three-dimensional velocity field of present-day crustal motion of the Tibetan Plateau derived from GPS measurements. Journal of Geophysical Research: Solid Earth, 118:1–11. Molnar, P. and Tapponnier, P. (1975). Cenozoic Tectonics of Asia: Effects of a Continental Collision. Science, 189:419–426. Molnar, P. (1988). A Review of Geophysical Constraints on the Deep Structure of the Tibetan Plateau, the Himalaya and the Karakoram, and their Tectonic Implications. Philosophical Transactions of the Royal Society A: Mathematical, Physical and Engineering Sciences, 326(1589):33–88. Molnar, P., England, P., and Martinod, J. (1993). Mantle Dynamics, Uplift of the Tibetan Plateau, and the Indian Monsoon. Reviews of Geophysics, 31:357–396. Moresi, L. (2015). Computational Plate Tectonics and the Geological Record in the Continents. SIAM News, 48:1–6. Ninomiya, Y. and Bihong Fu, B. (2016). Regional Lithological Mapping Using ASTER-TIR Data: Case Study for the Tibetan Plateau and the Surrounding Area. Geosciences 2016, 6(3), 39; doi:10.3390/geosciences6030039. Patriat, P. and Achache, J. (1984). India-Eurasia collision chronology has implications for crustal shortening and driving mechanism of plates. Nature, 311:615–621. Pusok, A. E. and Kaus, B. J. P. (2015). Development of topography in 3-D continental-collision models. Geochemistry, Geophysics, Geosystems, 16(5):1378–1400. Royden, L. H., Burch el, B. C., King, R., Wang, E., Chen, Z., Shen, F., and Liu, Y. (1997). Surface Deformation and Lower Crustal Flow in Eastern Tibet. Science, 276(5313):788–790. Tapponnier, P., Zhiqin, X., Roger, F., Meyer, B., Arnaud, N., Wittlinger, G., and Jingsui, Y. (2001). Oblique Stepwise Rise and Growth of the Tibet Plateau. Science, 294(5547):1671–1677.