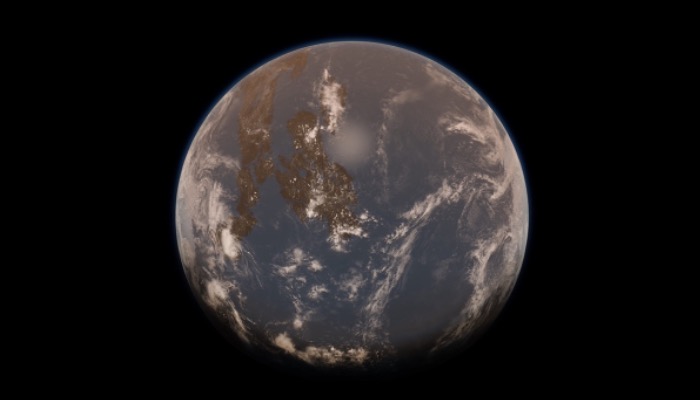
The question of how we came to be is closely tied with how the Earth became what it now is. What was the early Earth like? How did it evolve to become a habitable world? Given a fragmentary rock record, how can we investigate the early Earth and its evolution? Dr. Junjie Dong from Caltech writes about modeling the early Earth in a two-part blog. For the first part, he introduces the approach to early Earth questions and discusses how his work on Archean sea level and ocean volume suggests that the Archean Earth might have been a water world.
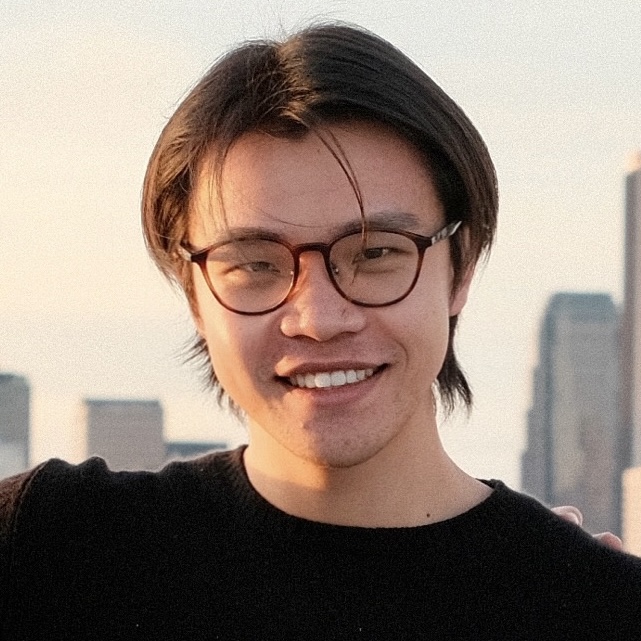
Dr. Junjie Dong is a Stanback Postdoctoral Fellow in Comparative Planetary Evolution (3CPE) at Caltech. He uses high-pressure experiments, thermodynamic modeling and first-principles simulations to study the early Earth and planetary interiors.
The Early Earth Questions
In my graduate school, one of the most popular reading groups in the department focused on the early Earth, discussing questions such as the initiation of plate tectonics and the origins of the dynamo among others. As a young graduate student, I was both excited and intimidated to partake in these weekly discussions with people like Nadja Drabon, Roger Fu, Andy Knoll, and Charlie Langmuir. I felt like a young puppy among seasoned dogs—curious and eager, but prone to the missteps typical of a novice. I vividly remember the aftermath of each heated discussion: I would leave either dejected, convinced that the questions about the early Earth were unsolvable due to the paucity of rock samples and their biased interpretations, or elated, believing that we could solve them by trusting a few select geological proxies and their idiosyncratic interpretations. These extremes of emotion were fleeting, and quickly overshadowed by new evidence and arguments the following week.
The approach to these early Earth questions was foreign to me at first. In my field of mineral physics, we rely heavily on strong empirical evidence. For example, we measure the sound velocity of a mineral and compare it to seismic velocities to infer the composition of the mantle (Anderson, 1972). The challenges in mineral physics are often technical, rooted in our ability to obtain reliable data (Fei & Walter, 2022), which made me an empiricist by necessity. In contrast, to explore the early Earth, one often has to work with under-constrained theoretical models and sometimes even metaphysical thought experiments due to the sparse and highly weathered rock record. Terms like “theoretical” and “metaphysical” may seem “unscientific” to some, but I do not share this Popperian skepticism1. On the contrary, I find these theoretical exercises profoundly fruitful. By idealizing the early Earth in often simple theoretical models, we gain access to its nature and a path to deeper understanding.
Here, rather than trying to comment on the specifics of geodynamic modeling practice, I would like to consider a less explored angle: how we idealize the early Earth in modeling, and what these exercises teach us. I will now use an example from my own work to illustrate the heuristic value of early Earth models. My views are certainly rudimentary, but the goal is to get the reader thinking about the methodology of modeling the early Earth.
Archean Earth was a Water World
In my 2021 paper published in AGU Advances, we estimated the size of the surface ocean in the Archean using a mantle water storage capacity argument (details of which can be found in Dong et al., 2021). We found that the Archean oceans might have been up to twice as large as the present-day ocean (Figure 1). This naturally led us to wonder how a larger early ocean might have altered the configuration of the Earth’s surface, potentially resulting in a landscape drastically different from today’s Earth.
For those working on the classic post-glacial sea level problem, modeling Archean sea level may seem like an impossible task. Accurately modeling post-glacial sea-level change requires knowledge of glacier dynamics, the Earth’s gravitational field, and how the Earth deforms under loading and unloading, combined with detailed oceanographic, meteorological, and coastline records (Mitrovica et al., 2011), about which we know almost nothing for the early Earth. However, post-glacial rebound research aims to understand changes to the accuracy of meters in order to understand sea level response over much shorter time scales. Our goal was different. We were not looking for the exact sea level, which is extremely difficult to estimate even for the Proterozoic and virtually unattainable for the early Earth. Instead, we were only interested in the relative configuration of mountains and seas and, ultimately, whether the early continents were flooded with water–critical to understanding the origin of life and the physical conditions that supported it.
In seeking a simple model to describe the solid Earth’s influence on sea level change over billions of years, we settled on a continental freeboard model based on isostatic balance, constructed by Flament et al. (2008) and later improved by Korenaga et al. (2017) and Rosas & Korenaga (2021). The isostatic model is used to relate the densities and thicknesses of different layers of the Earth’s surface to the sea level, including continental crust, oceanic crust, lithospheric mantle, and asthenospheric mantle (Figure 2).
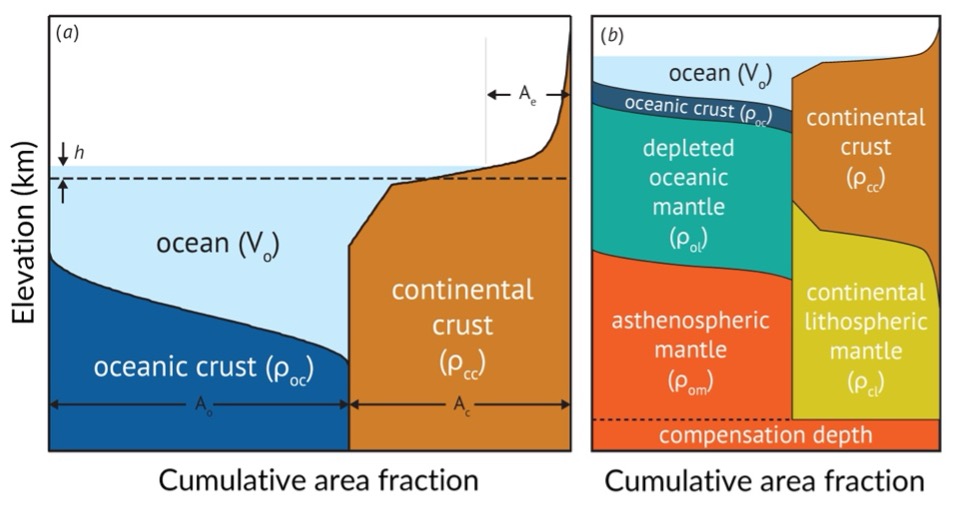
Figure 2 Schematic of continental freeboard modeling of the Archean Earth. Figure adapted from Korenaga et al. (2017).
Our findings (Dong et al., 2021, Figure 3) suggest that if the early oceans were more than twice their current volume, the continents were likely covered entirely by water, with only a few subaerial landmasses from hotspot volcanism or oceanic plateaus. If the early ocean was 1–2 times the present-day volume, the continents would still be largely flooded. With a fixed ocean volume, increasing the size of the early continental crust or decreasing its topography increases the flooded fraction.
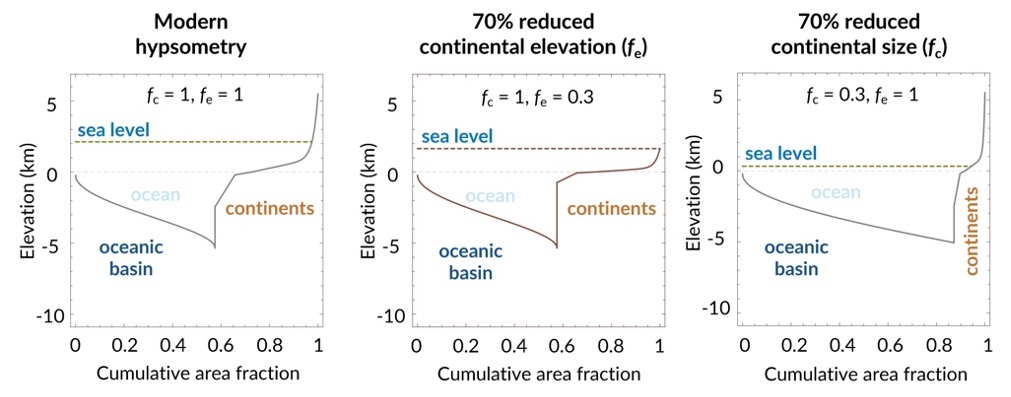
Figure 3 Flooding of the Archean Earth with various surface configurations; figures based on the results of Dong et al. (2021). Abbreviation: fc: fraction of the present-day continents; fe: fraction of the present-day elevation.
In this work, we sought to understand how the isostatic balance adjusts in response to a different ocean volume. While our work provides a new constraint for one parameter in the model–the ocean volume; the rest remains largely unconstrained. Extrapolating the isostasy model to the early Earth involves adjusting parameters such as the thermal and chemical buoyancy effects on the oceanic and continental lithosphere, as well as the hypsometric curve to reflect changes in emerging continental crust (Flament et al., 2008; Korenaga et al., 2017; Dong et al., 2021; Rosas & Korenaga, 2021). We know practically nothing about the validity of these parameter choices, and our parameterization, at best, is educated guesses and might well be incorrect. So why is modeling the early Earth still a useful exercise? In the second part of this blog, I present some conceptual resources and attempt to answer this question.
1Popper proposed the so-called “Demarcation Problem”, in which one can distinguish between science and pseudoscience by falsifying hypotheses with critical evidence; a falsifiable hypothesis would be considered scientific (Popper, 2002).
References Anderson, O. L. 1972, Tectonophysics, 13, 521, doi: 10.1016/0040-1951(72)90036-4 Dong, J., Fischer, R. A., Stixrude, L. P., & Lithgow-Bertelloni, C. R. 2021, AGU adv., 2, e2020AV000323, doi: 10.1029/2020AV000323 Fei, Y., & Walter, M. J., eds. 2022, Static and Dynamic High Pressure Mineral Physics, 1st edn. (Cambridge University Press), doi: 10.1017/9781108806145 Flament, N., Coltice, N., & Rey, P. F. 2008, Earth Planet. Sci. Lett., 275, 326, doi: 10.1016/j.epsl.2008.08.029 Korenaga, J., Planavsky, N. J., & Evans, D. A. D. 2017, Phil. Trans. R. Soc. A., 375, 20150393, doi: 10.1098/rsta.2015.0393 Mitrovica, J. X., Gomez, N., Morrow, E., et al. 2011, Geophys. J. Int., 187, 729, doi: 10.1111/j.1365-246X.2011.05090.x Popper, K. R. 2002, Conjectures and Refutations: The Growth of Scientific Knowledge (Routledge) Rosas, J. C., & Korenaga, J. 2021, Nat. Geosci., 14, 51, doi: 10.1038/s41561-020-00673-1