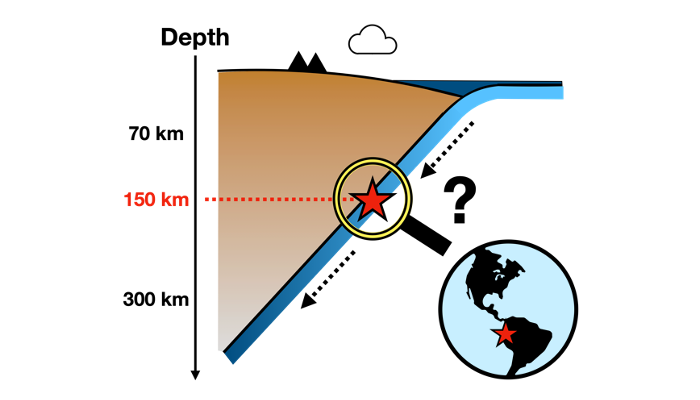
Most earthquakes on Earth start in the shallow, brittle part of the planet. However, there are several regions where earthquakes happen deep in the mantle. Where are these regions? Why do these earthquakes get so deep? In this week’s blog post, Ayako Tsuchiyama from the Massachusetts Institute of Technology (MIT) takes us on a journey into the mysterious world of deep earthquakes.
Growing up in Japan where large earthquakes frequently occur due to the location near plate boundaries, I was motivated to understand their physical mechanism and explore the possibility of earthquake prediction to save our lives, society, and culture. The 2011 Tohoku earthquake occurred when I was in middle school and about to start thinking about what I would do for my life. After several years, I was fortunate to have an opportunity to listen to a lecture about earthquakes at Tohoku University, very close to the epicenter of the 2011 Tohoku earthquake. I was fascinated when one of the lecturers mentioned that deep earthquakes are one of the long-standing mysteries in seismology, posing challenges due to their greater depths of up to ~660 km and limited observations.
Deep earthquakes represent the frontier of subduction zones as the physical mechanisms are intricately tied to the history of subducting tectonic plates. These plates transport fluids and pre-existing faults to the deep Earth. The fluids and the faults play a crucial role in triggering earthquakes at depths where extremely high temperature and pressure conditions make brittle failure less likely. Beyond their significance in seismology, deep earthquakes provide crucial insights into the mantle convection processes in geodynamics and geochemistry. They shed light on how materials circulate within Earth, and potentially in other Solar planets, offering a window into the planet’s formation history.
While working on deep earthquakes for my master’s thesis, which was later published as Tsuchiyama and Nakajima (2022), we discovered multiple pairs and a triplet of deep earthquakes at ~350 km in the Pacific plate beneath central Japan. Despite their nearly identical locations, waveforms, and focal mechanisms, these earthquakes suggested different physical mechanisms. Typically, we assume that earthquakes with similar waveforms occur with similar focal mechanisms. Therefore, we expected those similar deep earthquakes to have nearly identical source parameters. However, our findings, the diversity of source parameters within similar deep earthquakes, implied that the physical mechanism of deep earthquakes can potentially vary even under equivalent temperature and pressure conditions.
What controls the physical mechanism of deep earthquakes? This question leads me to my Ph.D. work on intermediate-depth earthquakes within the Bucaramanga Nest in Colombia. An earthquake nest refers to a localized cluster of earthquakes characterized by a higher seismic rate compared to the surrounding region, and it persists for several years or longer. There are three such nests of intermediate-depth earthquakes globally: Vrancea in Rumania, Hindu Kush in Afghanistan, and Bucaramanga in Colombia (Prieto et al., 2012). The Bucaramanga nest in particular exhibits the highest concentrations of deep earthquakes, with a seismic rate of approximately seven events per day (M> 2.5) and a strange absence of large-magnitude events (M>6.5 or larger). In contrast, the other two nests have lower rates, and their seismic activity may temporarily increase following a large earthquake.
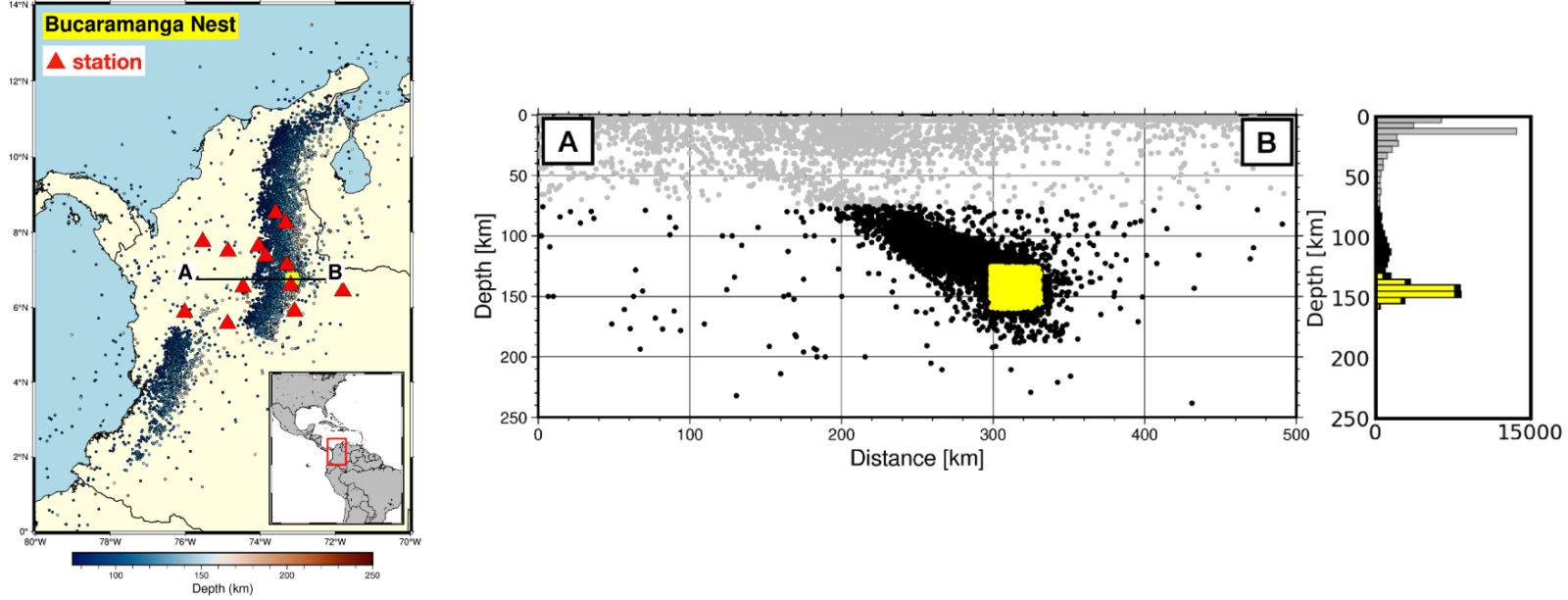
Seismicity in and around Bucaramanga Nest. Earthquake and station data from Servicio Geológico Colombiano. (Image Credit: Ayako Tsuchiyama)
To analyze how its seismic activity evolves through time, I started to explore the Bucaramanga Nest as a natural laboratory of deep earthquakes with the goal of creating an earthquake catalog from scratch. Employing template matching, I categorized deep earthquakes based on waveform similarity to gain insights into the underlying processes that transiently change either the source mechanism or surrounding medium over several years. We recently discovered a multi-year evolving process within a cluster of earthquakes sharing similar waveforms thanks to the extensive dataset, which short-term studies of large-deep earthquakes could not uncover. Since this is a very preliminary result, we are currently validating this observation through precise relocation and measurement of structural properties around the source area.
My interest has recently extended beyond deep earthquakes, leading me toward the field of planetary seismology, where I aim to decipher mechanisms from earthquake signal records on other celestial bodies. Surprisingly, Frohlich and Nakamura (2009) suggest that deep moonquakes occur under conditions of temperature and pressure similar to those of intermediate-depth earthquakes on Earth! This discovery excited me, as it implied that my work on intermediate-depth earthquakes could be instrumental in understanding the interiors of other planets.
Subsequently, I embarked on my second project simulating river deltas on liquid lakes on Titan, Saturn’s icy moon partially covered with stable-surface liquid of hydrocarbons. This project is entirely distinct from my primary seismological work. NASA is planning to launch a new satellite mission for Titan called Dragonfly, which will collect seismic data that may provide insights into Titan’s interior, similar to recent observations of a molten silicate core on Mars through the InSight mission (Khan et al., 2023; Samuel et al., 2023). I am eagerly looking forward to the possibility of capturing evidence of deep “Titanquakes” in the coming decades!
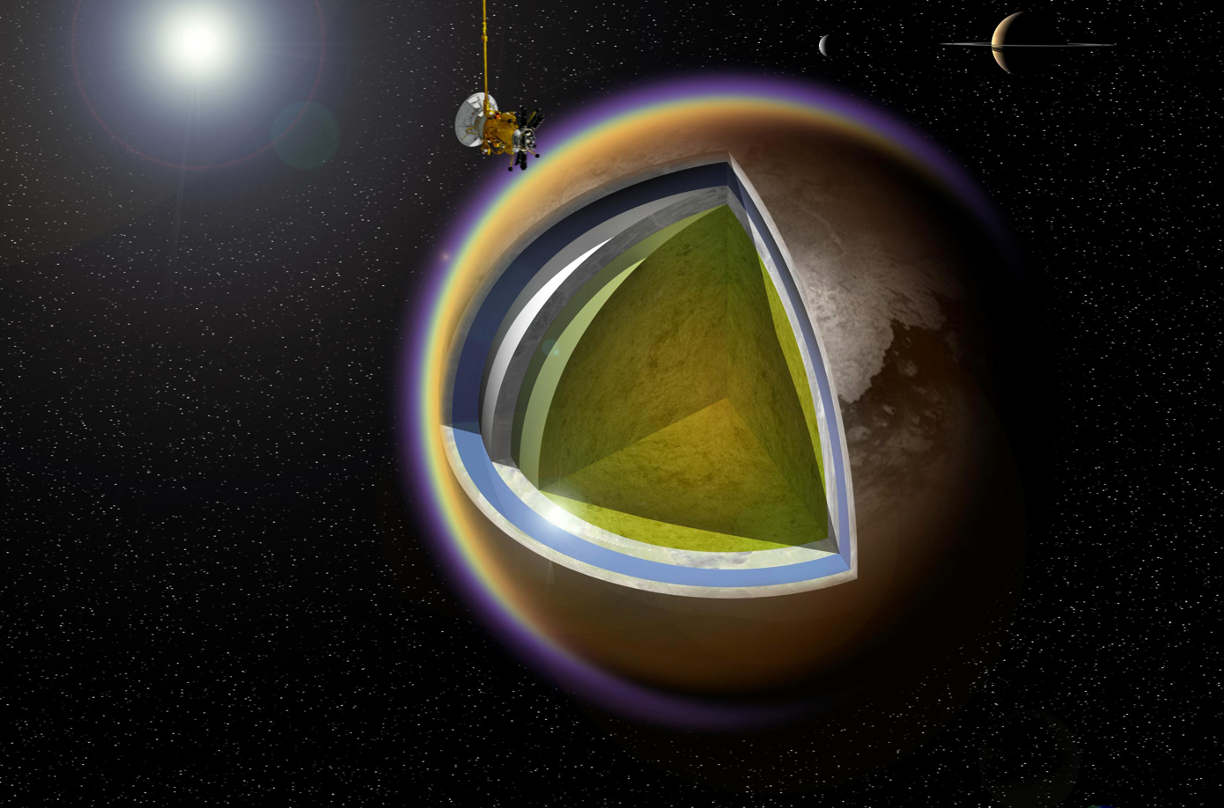
Artist’s concept of the internal structure of Titan. Ayako plans to study the seismic data from the “Dragonfly” mission to Titan that NASA will launch in the near future. (Image credit: A. D. Fortes/UCL/STFC)
Managing two distinct projects simultaneously has been still challenging for me, but thanks to the continuous support of my advisors, colleagues in both groups, researchers outside of MIT, and my family and friends, I have been fortunate to pursue these exciting projects and dream of potential discoveries about deep earthquakes on Earth and other planets.
References Frohlich, C., & Nakamura, Y. (2009). The physical mechanisms of deep moonquakes and intermediate-depth earthquakes: How similar and how different?.Physics of the Earth and Planetary Interiors, 173(3-4), 365-374. Khan, A., Huang, D., Durán, C., Sossi, P. A., Giardini, D., & Murakami, M. (2023). Evidence for a liquid silicate layer atop the Martian core.Nature, 622(7984), 718-723. Prieto, G. A., Beroza, G. C., Barrett, S. A., López, G. A., & Florez, M. (2012). Earthquake nests as natural laboratories for the study of intermediate-depth earthquake mechanics.Tectonophysics, 570, 42-56. Samuel, H., Drilleau, M., Rivoldini, A., Xu, Z., Huang, Q., Garcia, R. F., Lekić, V., Irving, J. C. E., Badro, J., Lognonné, P. H., Connolly, J. A. D., Kawamura, T., Gudkova, T., & Banerdt, W. B. (2023). Geophysical evidence for an enriched molten silicate layer above Mars’s core.Nature, 622(7984), 712-717. Tsuchiyama, A., & Nakajima, J. (2021). Diversity of deep earthquakes with waveform similarity.Physics of the Earth and Planetary Interiors, 314, 106695.
Michael Pons
Hi Ayako, really interesting, what mechanism do you propose for the Bucaramanga Nest deep earthquakes ?
Dehydration, slab bending, or maybe something else ? cheers.