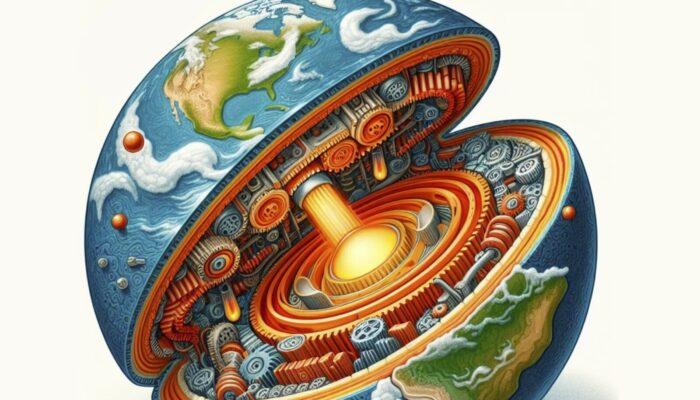
The Earth’s internal engine drives several geodynamic processes such as continental rifting and dynamic topography, shaping mesmerizing landscapes. Through numerical modeling, insights into formations like the Continental Rift of Southeastern Brazil deepen our appreciation of Earth’s dynamic nature and the forces sculpting its surface.
The heat engine beneath our feet
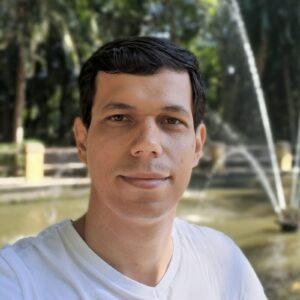
Rafael Monteiro da Silva is a PhD in geophysics from the Institute of Astronomy, Geophysics, and Atmospheric Sciences (IAG) at the University of São Paulo (USP). He specializes in numerical modeling of geodynamic processes, with a particular emphasis on crustal deformation and surface processes occurring along rifted margins, such as those found in South America.
Not so long ago, human history was marked by the steam engine, a symbol of the Industrial Revolution that had a revolutionary impact on our civilization. With the steam engine powering locomotives, humans gained a new way to traverse large distances, allowing us to appreciate mesmerizing landscapes. Yet, the formation and evolution of those landscapes are related to a different type of thermal machine: our planet Earth.
Formed 4.6 billion years ago from a blend of dust and gas surrounding a young Sun, Earth’s primordial heat and radioactive decay have controlled many geodynamic processes, including volcanic eruptions, intrusion of molten rocks, metamorphic processes, mantle convection and, ultimately, plate tectonics. Continental rifting, a major feature of plate tectonics, comprises the processes that break the continents apart, create two diverging oceanic plates along a ridge, and originate rifted margins. These rifted margins mark the limit between the oceanic and continental domains.
On a global scale and over a long term, rifts are key for shaping Earth’s topography. Changes in elevation are caused by the shifting and rising of land along the edges of the rift, reaching up to a couple of kilometers in height. Ongoing processes, such as erosion, relieve pressure on the lithosphere – the rigid, outermost shell of rocks of the planet –, and sustain uplift, flexure, and seismic activity for tens of millions of years (Buiter et al., 2023). The topographic variations can also occur due to the convective currents in the mantle, pulling up or down the Earth’s surface (Braun, 2010).
Thermomechanical numerical modeling and landscapes
In the past few decades, significant advancements have been made in computational geodynamics towards the understanding of the formation and evolution of divergent continental margins. Two distinct branches of numerical modeling approaches emerged for this purpose: landscape evolution models (LEMs) and thermomechanical models. LEMs simulate the evolution of landscapes by considering interactions between surface processes, such as erosion and sedimentation, as well as isostasy and lithospheric flexure. LEMs studies primarily concentrate on modeling the later phase of divergent margins after they have separated (van der Beek, 2013). Thermomechanical models, on the other hand, are designed to simulate lithospheric thinning from the onset of rifting. Both of these models offer valuable insights into the dynamic processes shaping divergent continental margins (Brune et al., 2023).
An example where thermomechanical numerical models helped to improve our comprehension between the inner connection of the Earth’s internal engine and the interplay of forces that shape our planet’s landscapes is the Continental Rift of Southeastern Brazil (CRSB). In this region, several basins within the continent are parallel to the margin, lying between a double pattern of cliffs formed by the Serra da Mantiqueira and Serra do Mar, product of tectonic activity which occurred nearly 60 million years after the opening of the South Atlantic ocean (Riccomini et al., 2004). While qualitative models have long been proposed to explain the origin of this continental rift, only recently a quantitative approach has been employed to test and validate these theories.
Based on numerical experiments that simulates the evolution of the lithosphere since the opening of the South Atlantic, around 130 million years ago, the CRSB formation has been found to be related to three main elements (Figure 1). These elements include the coupling between the crust and mantle, and the erosion of a large amount of rocks in the coastal area, due to the retreat of the Serra Mar escarpment. Moreover, preexisting crustal weakness zones in the interior of the continent favored tectonism during the postrift phase (Silva and Sacek, 2022).
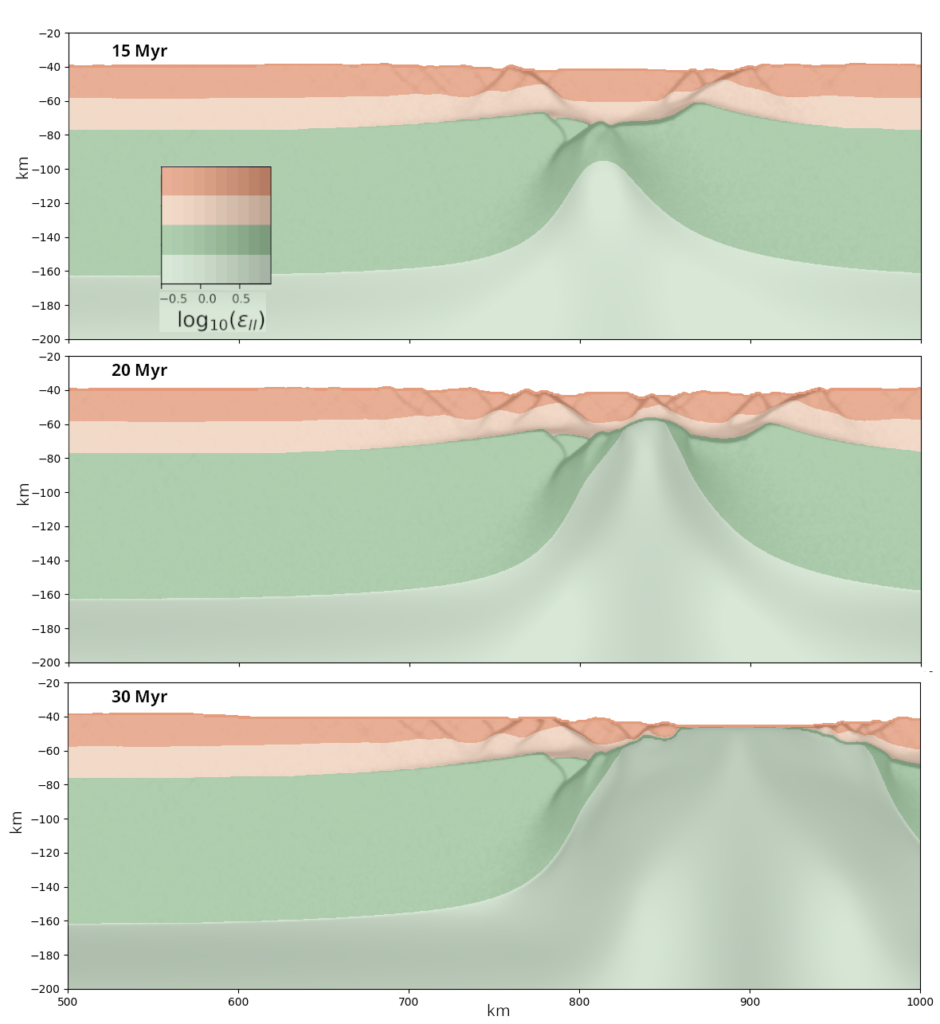
Figure 1: Evolution of lithospheric stretching and rifting depicted through a numerical scenario. The upper and lower crust are represented by dark and light orange colors, respectively, while the lithospheric and sub-lithospheric mantle are represented by dark and light green, respectively. Shades of gray indicate the magnitude of cumulative strain.
Mantle flow and the deflection of the Earth’s surface
Another process that results from Earth’s heat machine is the so-called dynamic topography. Caused by variations in temperature and density, convective movements in the mantle generate buoyancy forces that modify the surface (Figure 2). This dynamic topography leads to changes in elevation over time, shaping the Earth’s surface.
In places where flow rises towards the surface, the topography experiences uplift, that is, an elevation of the surface. On the other hand, in regions where the convective current sinks, it causes the topography to move downwards, generating a surface depression. The maximum amplitude of this type of topographic change can reach up to 1000 m height and extend from several hundred to several thousand kilometers. These variations in topography are transient in the geological time and proportional to the depth, vigor and scale of the mantle flow. Evidence for this process is found in the geological and geomorphological record, interpreted from the shifts in shorelines and studies on the formation of mountain ranges. These changes offer valuable insights into Earth’s past and present evolution (Braun, 2010).
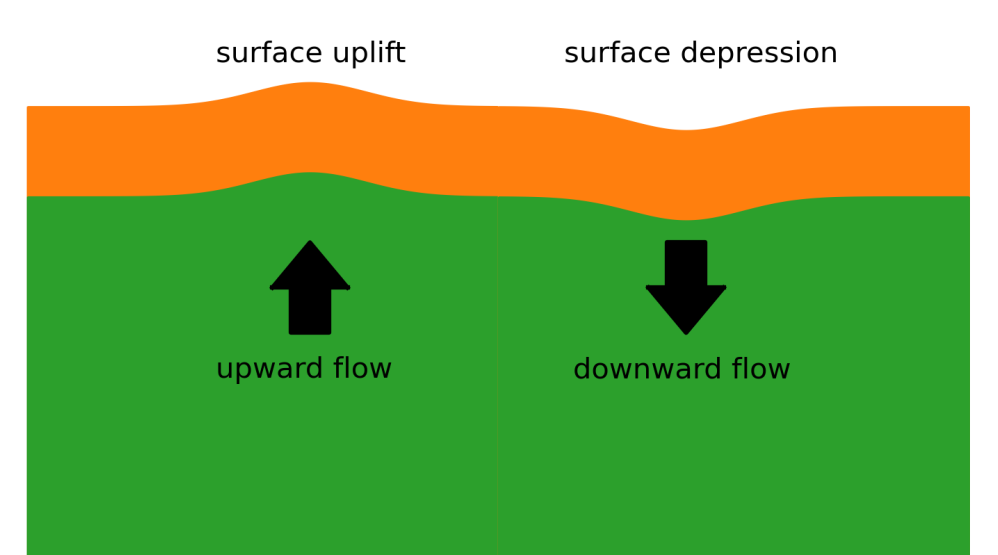
Figure 2: Simple sketch illustrating dynamic topography. Orange and green layers represent the lithosphere and asthenosphere, respectively. Arrows indicate the direction of the mantle flow which induces the uplift or depression of the surface.
The Earth’s internal engine has a broader complexity than the steam engine and is an ever-evolving system. The formation and evolution of the Continental Rift of Southeastern Brazil highlights the subtle interplay of forces that shape the landscape of our planet. The dynamic topography shows a key bond between mantle flow and surface vertical movements. Both serve as reminders that, while the Industrial Revolution relied on a relatively straightforward application of thermal energy, the Earth’s engine operates on a bigger, far more intricate scale. By understanding these processes, we gain a deeper appreciation for the dynamic nature of our planet and the forces that continue to sculpt its surface.
References: [1] Buiter, S. J., Brune, S., Keir, D., & Peron-Pinvidic, G. (2023). Rifting continents. In Dynamics of plate tectonics and mantle convection (pp. 459-481), Elsevier, https://doi.org/10.1016/B978-0-323-85733-8.00016-0 [2] van der Beek, P. (2013). Modelling landscape evolution. Environmental modelling: finding simplicity in complexity, 309-331, Wiley Online Library, https://doi.org/10.1002/9781118351475.ch19 [3] Brune, S., Kolawole, F., Olive, J. A. et al. (2023). Geodynamics of continental rift initiation and evolution. Nat Rev Earth Environ 4, 235–253, https://doi.org/10.1038/s43017-023-00391-3 [4] Riccomini, C., Sant’Anna, L. G., & Ferrari, A. L. (2004). Evolução geológica do rift continental do sudeste do Brasil. Mantesso-Neto, V.; Bartorelli, A.; Carneiro, CDR, 383-405 [5] Silva, R. M., & Sacek, V. (2022). Influence of surface processes on postrift faulting during divergent margins evolution. Tectonics, 41(2), e2021TC006808, https://doi.org/10.1029/2021TC006808 [6] Braun, J. (2010). The many surface expressions of mantle dynamics. Nature Geoscience, 3(12), 825-833, https://doi.org/10.1038/ngeo1020