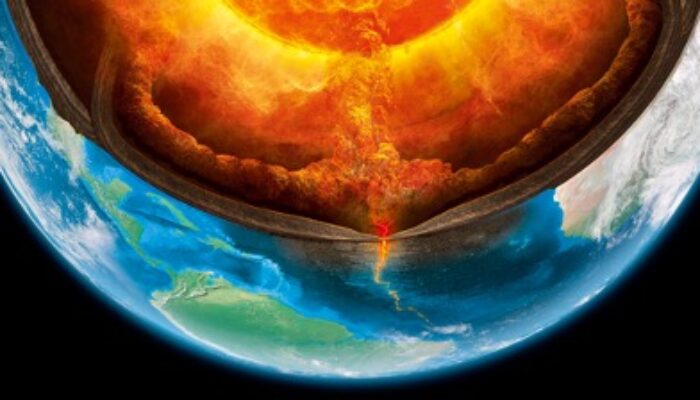
For understanding the dynamics and evolution of the Earth’s mantle, knowledge of mantle’s viscosity structure is very important. In this week’s blog, Kenneth Gourley from University of Arizona discusses the variation of viscosity structure in Earth’s mantle in regional scale.
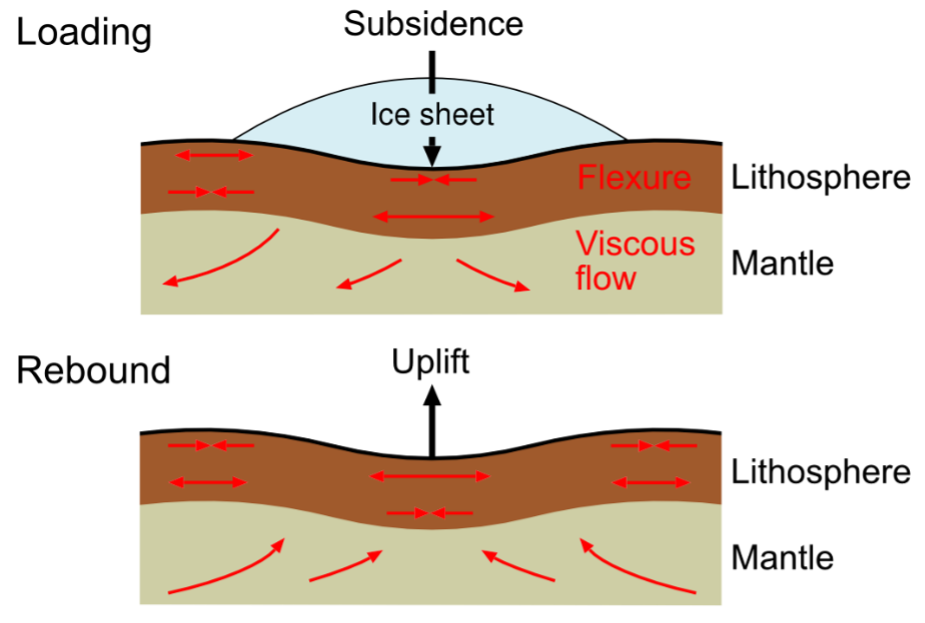
Figure 1. Illustration of the viscous response of the mantle and the lithosphere to the glacial loading (top) and subsequent unloading (bottom) from the last ice age to present day. The observations of this viscous response allows geodynamicists to constrain mantle viscosity. Reproduced from Dr. Volker Klemann, GFZ.
In order to understand the dynamics and evolution of the Earth’s mantle, geodynamicists model mantle flow using large, complex software suites (e.g. CitcomS, ASPECT, and Underworld). These programs solve the governing equations of fluid mechanics on a finite grid, allowing us to understand how mantle behavior changes as a function of various user inputs. The mantle behaves viscoelastically, where elastic deformation is reversible and occurs over very short timescales, while viscous deformation is permanent and occurs over much longer timescales. Therefore, accurate models of mantle convection through Earth’s history require reasonable estimates of mantle viscosity.
Measuring viscosity in the mantle is quite difficult due to the long timescales of deformation involved. The most accurate method would involve observing how quickly an object, such as a subducting slab, sinks through the mantle. Given that the mantle flows on the order of one centimeter per year, it would take over a million years for a slab to sink a distance we can currently resolve with seismic tomography. In the meantime, we can use constraints from the geoid and observations of glacial isostatic adjustment.
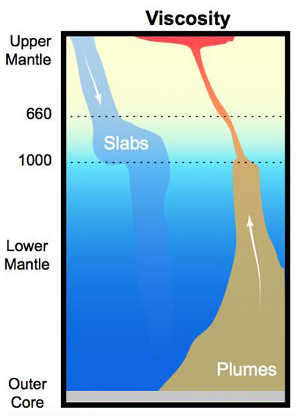
Figure 2. Illustration of the effect that a proposed viscosity jump in the mid-mantle has on subducting slabs and rising plumes. Higher density slabs stagnate and thicken above the viscosity jump, while lower density plumes stagnate and thicken below the viscosity jump. This viscosity jump being present at different depths in the mantle may explain regional variations in the depth that slabs and plumes stall and are deflected at, respectively. Modified from Drs. Nicholas Scmerr and Vedran Lekić, UMD.
A number of studies (e.g., Mitrovica and Forte 2004; Steinberger and Calderwood 2006) have produced models of global mantle viscosity as a function of depth. These radial profiles range over six orders of magnitude in many cases (from ~1018 to 1024 Pa·s) and tend to disagree with each other, especially in the mid-mantle which has the fewest constraints. They also neglect lateral viscosity variations, a concept that mantle geodynamicists have started to pay closer attention to in recent years.
Studies such as Lau et al. (2016) and Yang and Gurnis (2016) demonstrate that regional variations in viscosity impact the dynamics of mantle convection and lithospheric motion. These regional viscosity variations may also explain differences in the depth of slab stagnation beneath various subduction zones (Rudolph et al. 2015). Taking regional viscosity variations into account is especially important when examining short wavelength features of mantle convection. Unfortunately, including 3D viscosity variations in geodynamical models can be computationally expensive.
For my master’s thesis, I performed Bayesian inversions of mantle viscosity to better understand regional viscosity variations beneath southern Africa. The inversions explored a range of whole-mantle tomography models and were computed for both global and regional mantle domains. Overall, the regional models of mantle viscosity suggested that the mid-mantle is significantly stronger beneath southern Africa than compared to the global average. I am currently studying regional viscosity variations present in other parts of the mantle to determine what effect they have on processes such as dynamic topography, the stability of large low-shear-velocity provinces, and glacial isostatic adjustment.
References: Lau, H. C. P., J. X. Mitrovica, J. Austermann, O. Crawford, D. Al-Attar, and K. Latychev (2016), Inferences of mantle viscosity based on ice age data sets: Radial structure, Journal of Geophysical Research: Solid Earth, 121, 6991-7012 Mitrovica, J. X. and A.M. Forte (2004), A new inference of mantle viscosity based upon joint inversion of convection and glacial isostatic adjustment data, Earth and Planetary Science Letters, 225, 177-189 Rudolph, M. L., V. Lekić, and C. Lithgow-Bertelloni (2015), Viscosity jump in Earth’s mid mantle. Science, 350, 1349-1352 Steinberger, B. and A. R. Calderwood (2006), Models of large-scale viscous flow in the Earth's mantle with constraints from mineral physics and surface observations, Geophysical Journal International, 167, 1461–1481 Yang, T. and M. Gurnis (2016), Dynamic topography, gravity and the role of lateral viscosity variations from inversion of global mantle flow, Geophysical Journal International, 207, 1186–1202