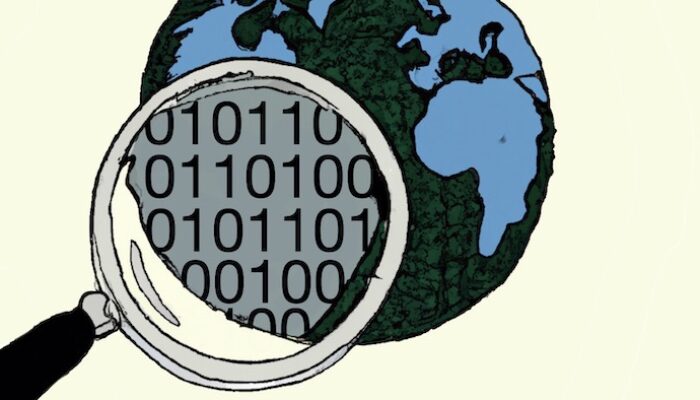
Within the vast realm of geoscience, the intricate mechanisms governing Earth’s internal processes continually pose challenges for researchers attempting to unveil its mysteries. This blog post acknowledges the inherent limitations of geological and geophysical data while highlighting the important role that geodynamic modeling plays in bridging these datasets. The motivations for this discussion arise from skepticism expressed during scientific events about this discipline, and–hopefully–this text can give more confidence to the power and capabilities of geodynamic modeling in enriching our understanding of Earth’s geological complexities.
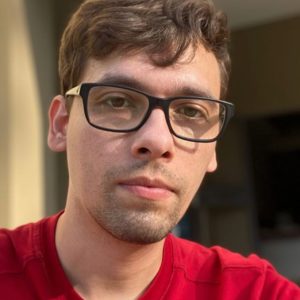
Jamison Assunção is a PhD student from Universidade de São Paulo currently researching about the Peruvian flat-slab subduction with numerical models.
There is only one Earth
There is only one Earth. Despite the glaringly obvious nature of this statement, its implications pose a significant challenge for geoscientists in their careers. The reason for this challenge lies in the absence of practical experiments to validate many of the hypotheses we have regarding the inner workings of our planet. Our understanding of the solid Earth is constrained by the lack of comparative data from other rocky planets, the technological difficulties in replicating extreme pressures and temperatures in the laboratory, and the relatively short duration of observations and measurements compared to the millions and billions of years of evolution. It could be argued that Geosciences deal with one of the highest number of hypotheses to test, which compels them to venture beyond the boundaries of what is already known through empirical evidence and traditional scientific methods.
The Challenges of Earth’s Complexity
Geoscientists have essentially two options in their quest for a deeper understanding of the solid Earth dynamics [1]. Option 1 involves studying the directly-observable geometry and composition of the rock record, within a thin shell of only a dozen kilometers (reached by the deepest drilling hole). In some cases, deeper materials are brought to the surface due to tectonic, volcanic and surface processes. However, even though the Earth is an 6371-km-radius spheroid, the remaining ~ 6359-km rock column remains mostly out of reach. These rocks can have ages spanning billions of years, and become harder to find and examine the older they are. Option 2 involves focusing on the present state of the Earth using indirect methods, primarily relying on the travel-time of seismic waves, and variations in gravity and magnetic fields. By adopting this approach, the study of the Earth’s structure becomes feasible across its vast entirety, with a setback that the data is less abundant and reliable for greater depths.
It is important to note, however, that integrating the two options previously mentioned presents a challenging obstacle. This is because we are unable to access rocks at great depths that span various ages, and we cannot utilize indirect methods to map the Earth’s structure in the past. Considering all this, numerical simulations arose as immensely powerful tools for comprehending aspects of our planet’s dynamics, providing us with new viewpoints through geodynamic modeling. Geodynamic modeling has faced a lot of skepticism regarding its applications, which the author of this piece has personally encountered in various events, including scientific conferences. While scientific skepticism is a natural and constructive part of the scientific process, certain opinions appear arbitrary and disregard modeling as a valid source of knowledge for understanding the Earth. Failing to recognize its power and usefulness poses yet an additional barrier to tackling the complex challenges in Geosciences.
Additionally, some of the criticism directed towards numerical codes is entirely justified. The first issue refers to the user experience, making the code challenging to use. The second problem relates to the “black-box” factor, concealing from the user the underlying reasoning behind the physical and/or mathematical considerations, whether intentionally or inadvertently.
The Power of Geodynamic Modeling: connecting direct and indirect methods to study the solid Earth
Geodynamic modeling serves as a crucial bridge between the direct and indirect options, effectively connecting and reconciling them. The underlying concept is as follows: if there is a hypothesis regarding the Earth’s past structure, it can be simulated and tested to determine if it aligns with both surface observations and the current state of the Earth. By employing numerical simulations, it becomes possible to simulate millions of years within a matter of hours. Although not equivalent to physical experiments, this approach holds immense value in quantifying and assessing our existing understanding while also exploring alternative explanations.
These models, rooted in our physical and mathematical comprehension of the world, offer means to expose gaps in our understanding of the underlying processes or deficiencies in their formulation. Therefore, numerical models play a vital role in the field of geosciences and should not be overlooked or disregarded, but rather embraced as a valuable tool.
Inspiration and Validation: the Calabrian subduction zone
Numerical models not only offer confirmation of our direct and indirect observations but also provide valuable insights into the evolutionary processes of our planet, often leading to the generation of new ideas based on quantitative information. A noteworthy recent illustration of this can be found in the work conducted by Gallen et al. (2023) [2]. The team’s research specifically focuses on the explanation of the Calabrian forearc uplift (located in Italy) in the last 30 Ma, exemplifying the capabilities and potential of numerical modeling in shedding light on complex geological phenomena.
In this work, the analysis of the forearc uplift history reveals intriguing patterns. The long-term uplift history displays a rapid uplift phase that gradually declines from 30 to 15 million years ago (Ma), followed by a period of slow uplift until 1.5 Ma. Finally, there is another phase of rapid uplift that continues to the present day. This pattern presents a challenge to our comprehension of crustal accretion processes, subduction velocity, and rock uplift resulting from plate interaction in a subduction zone.
According to the orogenic wedge theory [3] (see the blog post we made on Platt, 1986), the subduction wedge is governed by crustal accretion processes, leading to a positive correlation between relative plate subduction velocity and forearc rock uplift. In other words, the increased sediment flux should result in higher forearc buildup, reduced coupling at the subduction interface, and a faster velocity of the subducting plate. Surprisingly, studies have shown that the enlarged forearc surface in contact with the subduction interface may lead to increased frictional coupling between the plates, causing a decrease in the subducting plate’s velocity [4].
However, the observed uplift history in the Calabrian arc does not align with the expected uplift evolution based on the subduction rate observed in the region. As the slab freely descends, the uplift velocity should steadily increase until it reaches its maximum sinking speed due to its buoyancy relative to the ambient mantle. Nevertheless, when reaching the mantle transition zone, the slab’s velocity should decelerate due to the increasing viscosity of the surrounding mantle, eventually leading to stagnation.
This apparent inconsistency between uplift history and subduction velocity highlights the opportunity of numerical modeling in providing a trustworthy means to investigate and explain features that are otherwise inaccessible. By utilizing these models, researchers can explore alternative mechanisms and factors that could contribute to the observed uplift patterns, leading to new avenues of understanding the complex dynamics of the Earth’s geology.
Previous numerical models have demonstrated that the observed uplift pattern, as seen in the Calabrian forearc, can occur in subduction systems where the trench is retreating [5]. In this case, the interaction between the mantle corner flow above the descending plate and the mantle transition zone induces a trenchward movement of the upper plate, leading to the gradual subsidence of the forearc region. This process continues until the descending slab reaches the mantle transition zone, which coincides with the time when the forearc starts uplifting at 1.5 Ma. From these relationships, the authors hypothesize that the forearc uplift evolution results from the deep interaction between the slab and the surrounding mantle.
The consistency between the numerical models and the uplift history holds immense importance in understanding the surface expression arising from plate interaction with the mantle transition zone. In my view, we are heading in the right direction to achieve a deeper understanding of the Calabrian forearc uplift, thanks to these promising findings.
The implications of the findings in the Calabrian system and the use of numerical models extend beyond the study of this particular region. These findings can be a great source to constrain new studies focussing on the slab mantle transition zone interaction and its surface expression by building a tailored geodynamic model of the region. This, in turn, helps us to understand the complex geological processes at play in the Calabrian subduction zone, which could be also relevant for other subduction systems.
The growing availability of open-source geodynamic codes with complex physics, and increasing computational power allows the integration of geological and geophysical data into numerical models. These models are helping us to bridge the gap between conceptual models and data by providing physics-based solutions.
Pushing the boundaries with geodynamic models
The pursuit of understanding Earth’s complex workings poses unparalleled challenges for geoscientists. The unique nature of our planet, the limitations of direct experimentation, and the vast timescales involved necessitate innovative approaches. Geodynamic modeling emerges as a powerful tool, enabling the simulation of Earth’s past structures and validating observations against the current state of the planet. While numerical simulations provide valuable insights and inspire new avenues of research, they should always be complemented by other data to ensure a comprehensive understanding. By navigating these challenges and embracing the possibilities offered by geodynamic modeling, geoscientists continue to push the boundaries of knowledge, shedding light on the enigmatic processes that have shaped our remarkable planet.
References: [1] Gerya, T. (2014). Precambrian geodynamics: concepts and models. Gondwana Research, 25(2), 442-463. https://doi.org/10.1016/j.gr.2012.11.008 [2] Gallen, S.F., Seymour, N.M., Glotzbach, C. et al. Calabrian forearc uplift paced by slab–mantle interactions during subduction retreat. Nat. Geosci. 16, 513–520 (2023). https://doi.org/10.1038/s41561-023-01185-4 [3] Platt, J. P. (1986). Dynamics of orogenic wedges and the uplift of high-pressure metamorphic rocks. GSA Bulletin, 97(9), 1037–1053. https://doi.org/10.1130/0016-7606(1986)97<1037:DOOWAT>2.0.CO;2 [4] , , , , , , & (2021). The role of sediment accretion and buoyancy on subduction dynamics and geometry. Geophysical Research Letters, 48, e2021GL096266. https://doi.org/10.1029/2021GL096266 [5] , & (2020). Overriding plate velocity control on surface topography in 2-D models of subduction zones. Geochemistry, Geophysics, Geosystems, 21, e2019GC008900. https://doi.org/10.1029/2019GC008900