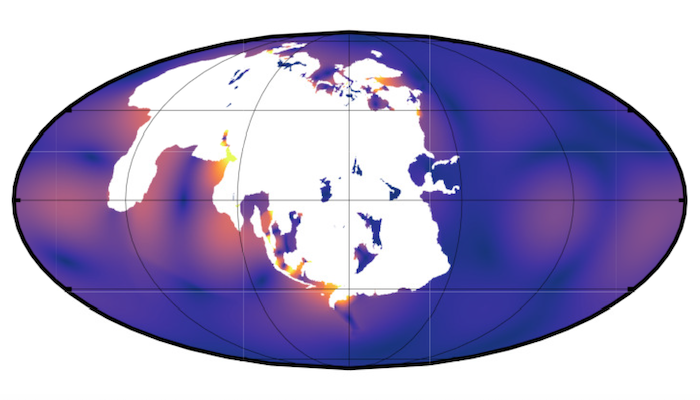
From orbital, to climatic, to tectonic evolution, many aspects of the Earth’s dynamics feature high degrees of cyclicity and episodicity, which can give us important insights into how the Earth’s works. The supercontinent cycle is an example capturing the Earth’s grandest scales and this week EGU geodynamics blog editor Tobias Rolf takes a closer look at it.
Pangaea. This is a familiar term to everyone who had introductory classes in the geosciences. It gives a name to the most recent assembly of the Earth’s continental landmasses, often referred to as a ‘supercontinent’. Its breakup set the pace for continental drift and plate tectonics during the last 200 Myr – a true landmark in the Earth’s evolution. However, a key question is whether the supercontinent Pangaea is unique or whether there have been other supercontinents. The Earth features cyclic/episodic behaviour of many flavours. Examples for such periodicity are the Milankovitch cycle which links Earth’s orbital to long-term climatic evolution, but also episodic reversals of the magnetic field and of course the Wilson cycle, which describes the opening and closing of ocean basins, such as the Atlantic. So why not also for supercontinents? In contrast to the Wilson cycle, the supercontinent cycle, originally proposed by Worsley et al. (1984), would operate on a global (not basin) scale with impacts even more striking and remarkable if the cycle took place.
And yes, there is support of such an episodic behaviour indeed, from the age spectra of zircons (Figure 1). Such spectra reveal pronounced peaks with the idea that each peak corresponds to a phase of intense continental collision, and thus the amalgamation of a supercontinent. During supercontinent maturity and break-up, however, continental crust production is expected to be reduced, reflected by a relatively low number of zircons of those ages. One could challenge that by arguing that the observed zircon spectra only contain what is preserved, and obviously ‘preserved = produced – recycled’ and not ‘preserved = produced’. So, the apparent peaks could just stem from periods when continental crust was better preserved. That’s a fair point and one example why the occurrence of (early) supercontinents is difficult to prove with the preserved geological record that is so strongly modified by plate tectonics since Pangea breakup. But, at least for this blog post, let’s trust what we have.
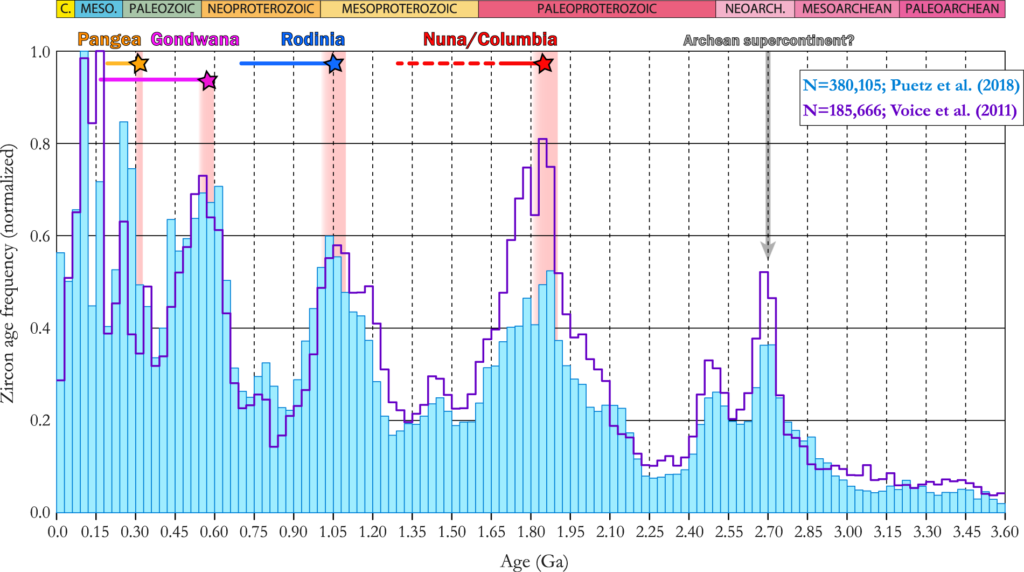
Frequency spectrum of preserved zircon ages using the data bases of Puetz et al. (2018) and Voice et al. (2011). Pink vertical stripes denote the estimated ages of major supercontinent assemblies. The lifetime of those continent clusters is indicated by the coloured horizontal bars. (Figure taken from Domeier et al., 2018.)
Gondwana, Rodinia, Columbia: these names often appear when supercontinents are discussed. Nuna, Pannotia, Kenorland: these may be less prominent names and – be aware – are sometimes used ambiguously to make it more confusing (e.g., Nuna = Columbia). A reason for such ambiguity may be a matter of definition – what is a supercontinent? What sounds like a simple question is actually quite difficult to answer. A cluster of a certain size? But what size? A cluster containing a certain number of previously dispersed fragments? But how many? One could think of various criteria, but none may be applied for all supercontinent clusters in the same manner.
Those clusters all featured different shapes, sizes (remember also that the Earth’s continental area has changed through time!), and the process through which they formed (e.g., Murphy & Nance, 2013). For example, Pangea likely formed via introversion, that is a new supercontinent forms at the location of its predecessor after closing the internal ocean that grew between the dispersing fragments. Thus, this is comparable to a global-scale Wilson cycle. Extroversion on the other hand features a new supercontinent in the opposite hemisphere of the previous one. The possibilities between these two idealised end-members are diverse (see e.g., Mitchell et al., 2012) as also supported by numerical modelling (Figure 2). To make things clearer – or not – one may even distinguish between supercontinents and megacontinents, where the megaversion is (obviously …) smaller than the superversion and could form in a pre-stage of completed assembly – something like present-day Eurasia (Wang et al., 2021).
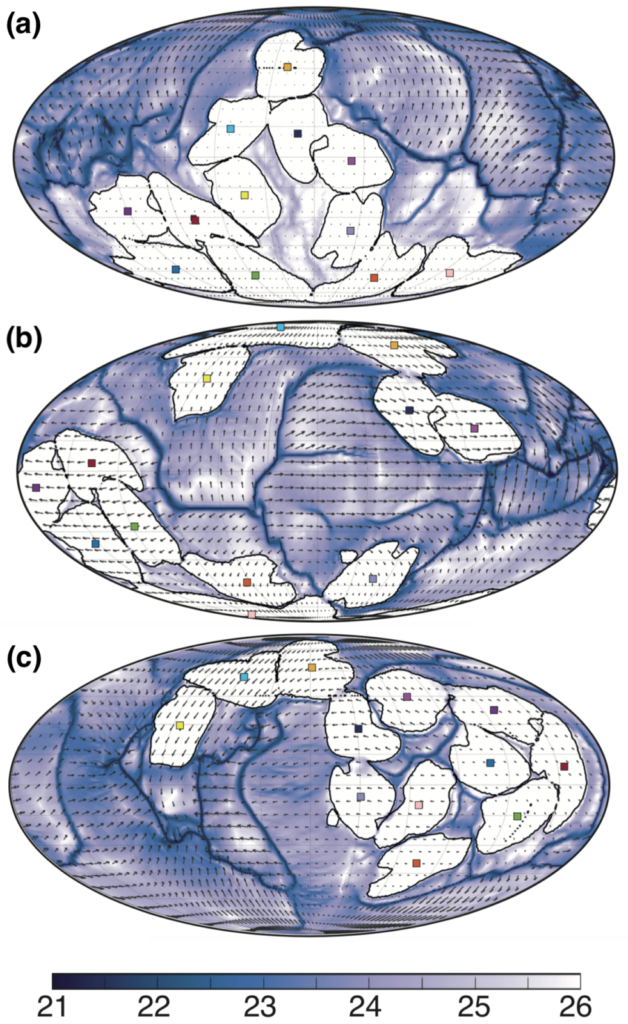
Figure 2 – Geodynamic model evolution featuring plate-like tectonic behaviour and capturing a supercontinent cycle: (a) Presence of a supercontinent A (model time: 1.52 Gyr), (b) Supercontinent A has fragmented by time 1.71 Gyr, (c) a new supercontinent B has formed by time 2.09 Gyr. Color coding: Decadic logarithm of viscosity. Continents (12 in total) are outlined in black. (Figure based on Rolf & Pesonen, 2018).
Alternatively, one could define a supercontinent as something that has a notable impact on the geodynamic evolution and whatever is coupled to this (Heron et al., 2020). If a continental cluster is large enough, heat may be trapped in the mantle below it, which could explain the emplacement of some large magmatic provinces such as CAMP (Central Atlantic Magmatic Province, Whiteside et al., 2010). Supercontinent formation could lead to large-scale reorganisation of mantle flow (O’Neill et al., 2009) and lead to changes in the layout of tectonic plates, thereby affecting the average age of the seafloor, the depth of ocean basins (Coltice et al., 2013), and sea level (Conrad, 2013). Supercontinents also matter for long-term climate and biogenic evolution: on an equatorial supercontinent, flora and fauna could flourish better than on a polar supercontinent. Also, an equatorial supercontinent may lead to different albedo, surface air temperature (including its latitudinal gradients), and precipitation distribution than a polar one. These processes all feedback with weathering, erosion and sedimentation, and thus eventually with tectonics. By reorganising the layout of plates and the distribution of subduction, supercontinents may also change the distribution of mass in the Earth’s mantle and induce True Polar Wander events. Such events lead to the readjustment of the Earth’s entire silicate shell with respect to the spin axis and are crucial for reconstructing the Earth’s paleogeography (see this recent blog post by Boris Robert).
After all, it is fair to state that large continental clusters – super-sized, mega-sized or just very large – could make major imprints on the evolution of the Earth. It is important to further understand these events of clustering to push our understanding of how plate tectonics operated in times prior to Pangea’s break-up when there is no seafloor preserved to reconstruct paleogeography. Numerical modelling provides relevant insights on supercontinent signatures on mantle and lithosphere (e.g., Rolf et al., 2012, Yoshida & Santosh, 2014; Heron et al., 2020). Yet model predictions seem quite sensitive to model assumptions such as mantle viscosity structure and the strength of oceanic and continental lithospheres. More exploration is needed.
Finally, the formation of supercontinents such as Pangea is a clear expression of the self-organisation of the Earth’s mantle and plate tectonics. The formation of a supercontinent may reorganise this system in such a way that its break-up and subsequent dispersion already induce the formation of a new supercontinent, although this may not be obvious in the immediate reorganisation happening alongside the break-up of the supercontinent. However, in a complex world like the Earth’s interior, many processes interact with each other, so it is not surprising that in the club of (potential) supercontinents, none is very similar to the other.
REFERENCES
- Coltice, N. et al., 2013, Convergence of tectonic reconstructions and mantle convection models for significant fluctuations in seafloor spreading, Earth Planet. Sci. Lett., 383, 92-100
- Conrad, C.P., 2013, The solid Earth’s influence on sea level, Geol. Soc. Am. Bull., 125, 1027-1052
- Davies, H.S., et al., 2020, Back to the future II: tidal evolution of four supercontinent scenarios, Earth Syst. Dynam., 11, 291-299.
- Domeier, M. et al., 2018, Episodic zircon age spectra mimic fluctuations in subduction, Scientific Reports, 8, 17471
- Heron, P. et al., 2020, Pannotia’s mantle signature: the quest for supercontinent identification, Geol. Soc. London. Spec. Pub., 503, 41-61
- Mitchell, R. et al., 2012, Supercontinent cycles and the calculation of absolute palaeolongitude in deep time, Nature, 2012, 482, 208-211.
- Murphy, B. & Nance, D., 2013, Speculations on the mechanisms for the formation and breakup of supercontinents, Geosci. Fron., 4, 185-194.
- O’Neill, C. et al., 2009, Influence of supercontinents on deep mantle flow, Gondwana Res., 15, 276-287
- Puetz, S. J. et al., 2018, Statistical Analyses of Global U-Pb Database 2017, Geosci. Fron., 9, 121–145.
- Rolf, T. et al., 2012, Linking continental drift, plate tectonics, and the thermal state of the Earth’s mantle, Earth Planet. Sci. Lett, 351-352, 134-146.
- Rolf, T. & Pesonen, L. J., 2020, Geodynamically consistent inferences on the uniform sampling of Earth’s paleomagnetic inclinations, Gondwana Res., 63, 1-14.
- Voice, P. J. et al., 2011, Quantifying the timing and rate of crustal evolution: global compilation of radiometrically dated detrital zircon grains, J. Geol., 119, 109–126).
- Wang, C. et al., 2021, The role of megacontinents in the supercontinent cycle, Geology, 49, 402-406
- Whiteside, J. et al., 2010, Compound-specific carbon isotopes from Earth’s largest flood basalt eruptions directly linked to the end-Triassic mass extinction, Proc. Nat. Academy Sci., 2010, 107, 6721-6725
- Worsley, T.R. et al., 1984, Global Tectonics and Eustasy for the Past 2 Billion Years, Marine Geology, 58, 373-400.
- Yoshida, M. & Santosh, M., 2014, Mantle convection modeling of the supercontinent cycle: Introversion, extrosversion, or a combination?, Geosci. Fron., 5, 77-82