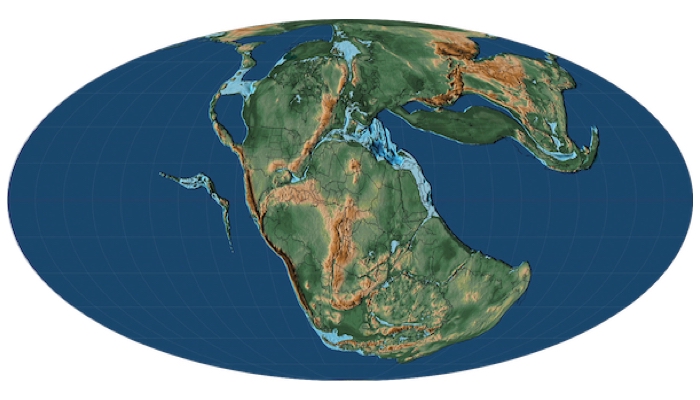
Paleogeography refers to the past distribution of landmasses and oceans and gives important insights into the state of previous tectonics. This is a useful cornerstone for many aspects of geodynamics and this week Boris Robert – previously postdoctoral fellow at the Centre for Earth Evolution and Dynamics Oslo (Norway) and currently visiting scientist at GFZ Potsdam (Germany) – tells us how we may try to solve this challenging puzzle, whose pieces move, reorient, and even change shape through time …
On geological scales, plate tectonics causes translation, convergence and divergence of plates. This creates an ever-changing geographic pattern of lands and oceans (Figure 1), and results in geological vestiges such as orogens, rifts and faults. Studying these relics allows us to reconstruct how plate tectonics shaped the Earth’s surface in the past. Paleomagnetic data is key for this and for making quantitative reconstructions of the past positions of continents. It uses the ancient magnetization preserved in surface rocks to define paleolatitude and -orientation of rigid continental blocks with respect to the Earth’s spin axis. Combining paleomagnetic and geological constraints can thus reveal how the Earth’s oceans opened and closed, how continents assembled into a supercontinent like Pangea, and how plate boundaries evolved through time. Such information is crucial for understanding the Earth’s tectonic and geodynamic history through deep time, and ultimately for interior evolution and dynamics!
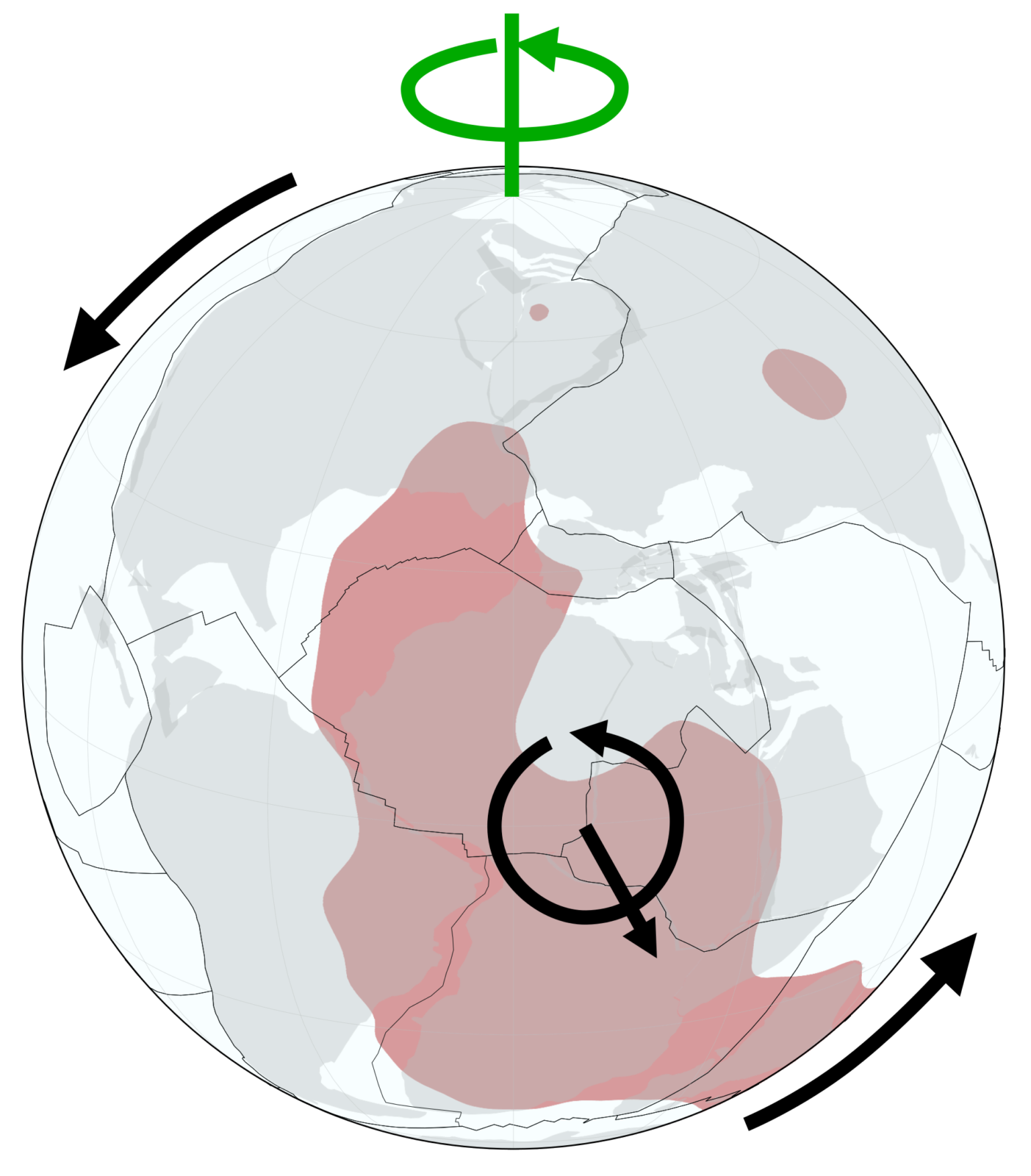
Figure 1: Example of a plate reconstruction and its link to deep mantle structure. This reconstruction is after Matthews et al. (2016) and Torsvik et al. (2019) at 110 Ma. Continents are shown in grey, plate boundaries in black, and the spin axis in green. One of the deep mantle’s large low shear velocity provinces (call them LLSVP, if you like) is shown in red (radially projected onto the surface). The black arrows depict the True Polar Wander event that occurred around 110 Ma [Credit: Boris Robert].
The lithosphere forms the skin of the evolving mantle and its fragmentation into plates of various size with different speeds is controlled by the coupling between the lithosphere and mantle flow. Because there is no direct observation of past mantle states and flow patterns, understanding mantle evolution may only be achieved by modelling the coupling between mantle dynamics and lithosphere using paleogeographic constraints as boundary condition. For example, the locations of subduction zones define subduction flux (e.g., Domeier and Torsvik, 2014) and thus the main downwelling flow in the mantle. Moreover, reconstructed hotspot locations give insight into where mantle plumes may have pierced the lithosphere. Nevertheless, interior evolution remains largely under-constrained, but at least available constraints have quality and robustness, haven’t they … ?
Well, reconstructing paleogeography becomes more and more difficult the further we go back in time, because the geological vestiges of past tectonic activity become more and more fragmentary. Ocean seafloor is no older than the Early Jurassic (~200 Myr ), so we are left with the continental crust to give as tectonic clues for times prior to that. Continental crust is better preserved, but the older its rocks are, the less are they exposed at the surface (e.g., Mooney, 2007). This results in less paleomagnetic data as possible sampling areas diminish. On top of that, older rocks are more likely re-magnetised during subsequent tectonic events, which results in reduced data quality and robustness. Uncertainties therefore grow and a continuous paleogeographic reconstruction of the Earth’s surface becomes more and more difficult to obtain.
But it is not hopeless, and a lot can still be learned from this approach! A key mechanism to better constrain paleogeography and its link to mantle dynamics is True Polar Wander (TPW). This mechanism corresponds to the coherent motion of the solid Earth (crust and mantle) with respect to the spin axis (Figure 2) and can be identified using paleomagnetism. It results from the conservation of angular momentum and is a consequence of changes in the distribution of mass heterogeneities inside the planet (Munk and McDonald, 1960). On geological timescales, the main heterogeneities are located in the mantle and stem from cold sinking slabs as well as from hot rising plumes. TPW is therefore intimately linked to mantle dynamics: its occurrence, style, and rates inform us about large-scale mantle flow.
Current mantle flow is dominantly quadrupolar and features two large upwellings rising from the base of the mantle encircled by a girdle of subduction (the “ring of fire”). The likely persistence of this pattern since at least 250 Ma stabilizes TPW, which dominantly results from rotations along an axis passing through the two large upwellings (Figure 1; e.g., Steinberger and Torsvik, 2008). For prior times, we actually know better about TPW than about mantle flow. Consequently, TPW can be a powerful indicator for the large-scale mantle flow in the past! Moreover, it can be used to constrain the paleolongitude of continents (e.g., Mitchell et al., 2017), a piece of information that individual paleomagnetic poles can typically not reveal. Therefore, analysis of TPW is an important task to better constrain paleogeography and mantle dynamics for deep times.
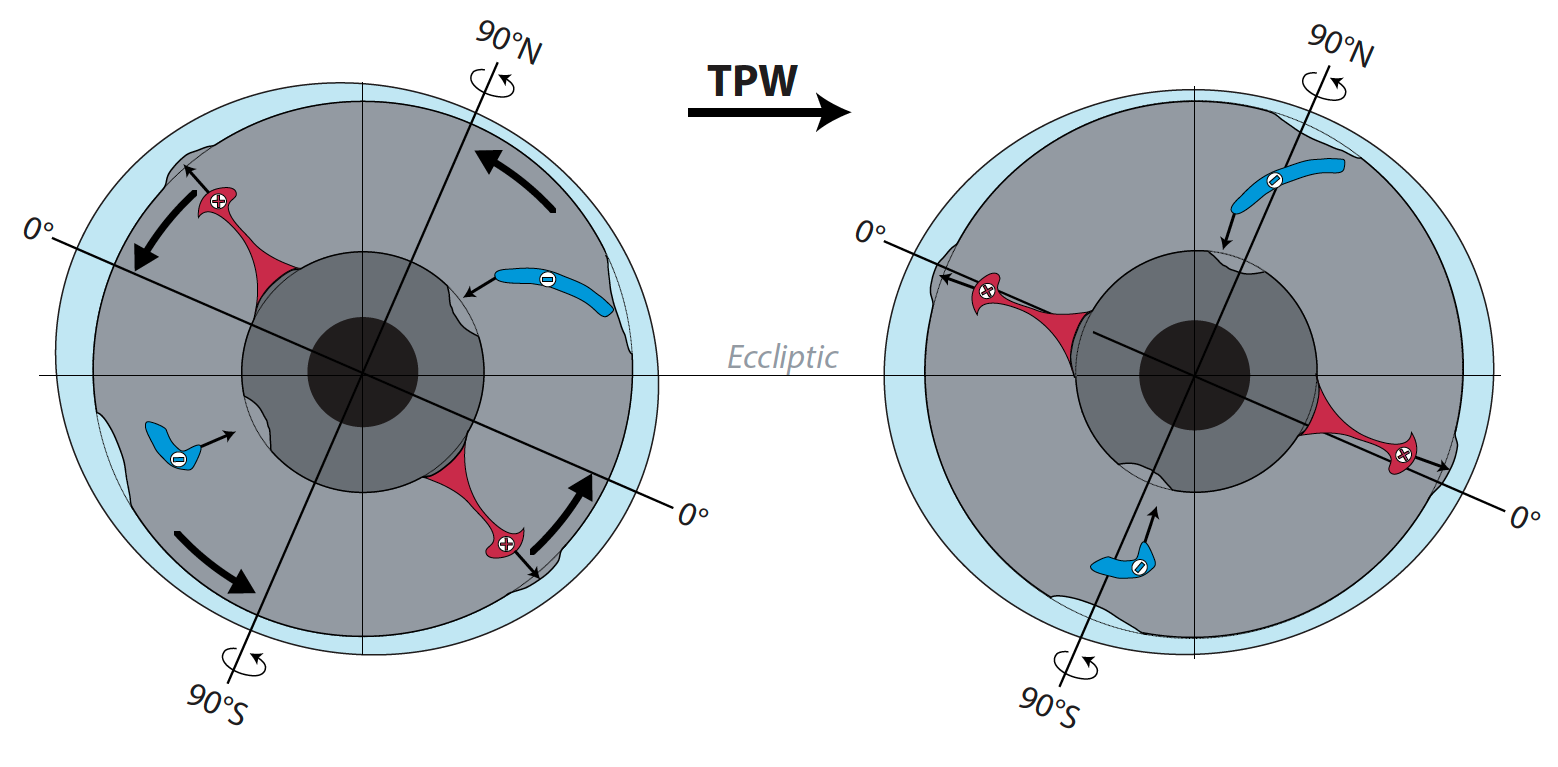
Figure 2: Conceptual illustration of True Polar Wander (TPW). The distribution of mantle mass anomalies induces a TPW event (fat black arrow). This is a rigid motion of the crust and mantle with respect to the spin axis that brings hot, light material (red) preferentially towards the equator and cold, heavy slabs (blue) closer to the rotation poles. Here conceptually simple, this is more complex in reality because the effect of mass anomalies on TPW changes with size and depth and as a function of the viscosity in the mantle. Note that TPW does not affect the position of the spin axis with respect to the ecliptic plane. [Credit: T. Rolf &B. Robert, based on Evans (2003)].
But determination of past TPW is not without difficulties! For the last 130 Myr, the situation is comparably comfortable as we can compare the reference frame constructed based on hotspot locations with the one constructed from paleomagnetic data to identify TPW reasonably accurately (e.g., Besse and Courtillot, 2002). The hotspot frame constrains the motion of plates with respect to the mantle and the paleomagnetic frame provides the motion of plates relative to the spin axis: combining the two therefore constrains the motion of the mantle with respect to the spin axis, that is TPW! Trouble starts before 130 Ma when the hotspot frame cannot be determined anymore. Then, TPW can still be inferred from paleomagnetic data, but with less certainty and only if its rate is significantly faster than the motion of individual plates. This is case for plates like Africa since Pangea breakup, for instance, that did not move much with respect to the mantle (e.g., Steinberger and Torsvik, 2008). During specific intervals of the Neoproterozoic (1000-541 Ma), TPW may also have been significantly faster than plate motions, so these are interesting periods to analyse (Maloof et al. 2006; Robert et al. 2017) and to learn more about the paleogeography and its relation to the large-scale interior structure of the Earth back then!
To sum up, paleogeography provides important constraints on past mantle dynamics. Paleomagnetism is central for this purpose as it constrains the rates of individual plate motions and of True Polar Wander. All this is needs to be linked to quantitatively constrain the evolution of the Earth’s lithosphere-mantle system!
Further reading
- Besse, J., & Courtillot, V. (2002). Apparent and true polar wander and the geometry of the geomagnetic field over the last 200 Myr. J. Geophys. Res. Solid Earth, 107, EPM-6.
- Domeier, M., & Torsvik, T. H. (2014). Plate tectonics in the late Paleozoic. Geosci. Front., 5, 303-350.
- Evans, D. A. (2003). True polar wander and supercontinents. Tectonophys., 362, 303-320.
- Maloof, A. C., Halverson, G. P., Kirschvink, J. L., Schrag, D. P., Weiss, B. P., & Hoffman, P. F. (2006). Combined paleomagnetic, isotopic, and stratigraphic evidence for true polar wander from the Neoproterozoic Akademikerbreen Group, Svalbard, Norway. Geol.Soc. America Bull., 118, 1099-1124.
- Matthews, K. J., Maloney, K. T., Zahirovic, S., Williams, S. E., Seton, M., & Mueller, R. D. (2016). Global plate boundary evolution and kinematics since the late Paleozoic. Global Planet. Change, 146, 226-250.
- Mitchell, R. N., Kilian, T. M., & Evans, D. A. (2012). Supercontinent cycles and the calculation of absolute palaeolongitude in deep time. Nature, 482, 208-211.
- Mooney, W.D. (2007), Crust and lithosphere—global crustal structure, in Seismology and the Structure of the Earth (ed. M. Kono). Treatise on Geophysics, vol. 1, chapter 11, 361–399, Elsevier, Amsterdam.
- Munk, W. H., & MacDonald, G. J. (1960). The rotation of the earth; a geophysical discussion. Cambridge Univ. Press.
- Robert, B., Besse, J., Blein, O., Greff-Lefftz, M., Baudin, T., Lopes, F., … & Belbadaoui, M. (2017). Constraints on the Ediacaran inertial interchange true polar wander hypothesis: A new paleomagnetic study in Morocco (West African Craton). Precambrian Res., 295, 90-116.
- Scotese, C.R. (2014), Atlas of Middle & Late Permian and Triassic Paleogeographic Maps, map 46 from Volume 3 of the PALEOMAP Atlas for ArcGIS, Mollweide Projection, PALEOMAP Project, Evanston, IL. (https:doi.org/10.13140/2.1.2609.9209)
- Steinberger, B., & Torsvik, T. H. (2008). Absolute plate motions and true polar wander in the absence of hotspot tracks. Nature, 452, 620-623.
- Torsvik, T. H., Steinberger, B., Shephard, G. E., Doubrovine, P. V., Gaina, C., Domeier, M., … & Sager, W. W. (2019). Pacific‐Panthalassic reconstructions: Overview, errata and the way forward. Geochem., Geophys., Geosyst., 20, 3659-3689.