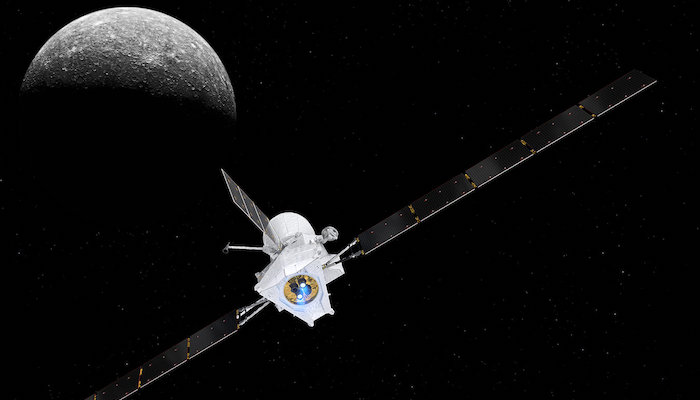
It is the smallest planet of the Solar System, the closest to the Sun and the quickest at orbiting around it, the one with the least inclined and most elongated orbit, the only one where a day lasts two-thirds of a full year, the one with the highest bulk density. Mercury is a planet of extremes, but rarely visited by space missions (compared to Mars, Venus and the Moon). This week Dr. Nicola Tosi, senior scientist at the Institute of Planetary Research of the German Aerospace Center (DLR, Berlin) tells us about the mysterious innermost planet and its future exploration.
Mercury sits deep in the Sun’s gravity well, which makes it difficult for an approaching spacecraft to slow down sufficiently to enter planet’s orbit. Following an idea of the Italian mathematician Giuseppe “Bepi” Colombo, in 1976, NASA’s Mariner 10 was the first space probe to reach Mercury and to do so by using the gravity of another planet — Venus. Mariner 10 was not designed to orbit Mercury though. It performed three flybys that provided the first close-up view of about half of the planet. The surface appeared old and highly cratered, to some extent similar to the Moon. Unexpectedly, Mariner 10 detected a global magnetic field – largely dipolar as the Earth’s, but much weaker in intensity – making Mercury the only rocky planet besides the Earth to possess a planetary-scale field today, generated in the deep, iron-rich core. It was not until 2011 that a second spacecraft reached Mercury. After multiple flybys at Earth, Venus and Mercury itself, the MErcury Surface, Space ENvironment, GEochemistry, and Ranging (MESSENGER)spacecraft was inserted into a polar, highly elliptical orbit around Mercury on March 18, 2011. Over the course of four years, MESSENGER revolutionised our understanding of the innermost planet (Solomon et al., 2018). However, it also left several unanswered questions that ESA’s double spacecraft BepiColombo, launched in 2018, will try to address.
Many of the discoveries made by MESSENGER bear on Mercury’s interior structure and geodynamic evolution. While it was known from Earth-based radar measurements of Mercury’s rotation that the planet’s core must be (at least partially) liquid (Margot et al., 2007), the actual core size was not precisely known. MESSENGER’s gravity measurements constrained the core radius to ~2000 km, implying a mantle only ~400 km thick, roughly 200 km less than previously thought (e.g., Rivoldini & van Hoolst, 2013). Surface images revealed that the planet witnessed a major volcanic resurfacing around 4 Ga, with lava that covered vast areas of the northern hemisphere. This formed smooth plains that also characterize large expanses within and around the main impact basins (Head et al., 2011). Partial melt produced by mantle convection, also influenced by the energy deposited at depth by large impacts, was responsible for these eruptions. Most of the main volcanic units were likely emplaced by ~3.5 Ga (Byrne et al., 2016). Afterwards, the planet’s activity declined — although there is evidence of small-scale volcanism that may have lasted until as recently as ~1 Ga — and the interior began to cool and contract. The most distinctive geological features of Mercury are so-called lobate scarps, linear or arcuate segments up to hundreds of kilometers long. These scarps are thought to represent the surface expression of thrust faults that cut deep into the crust and formed as the planet shrank upon cooling causing the brittle crust to fracture. MESSENGER’s high-resolution images allowed the identification of a myriad of previously undetected compressive landforms whose cumulative length indicates that Mercury’s radius decreased by up to 7 km over the planet’s evolution (Byrne et al., 2014).
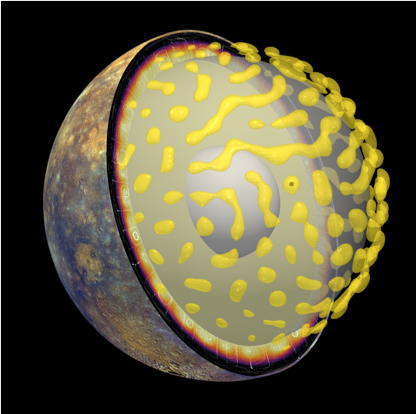
Fig. 1: Models of Mercury’s interior evolution satisfy various constraints such as the total amount of radial contraction associated with cooling of the interior and recorded by surface tectonic features, or the duration and extent of volcanism inferred from surface images. Significant uncertainties remain though. In Mercury’s thin mantle, the pattern of convection is characterised by regularly-spaced cells with small-scale down- and upwellings (yellow contours) that cannot be easily reconciled with large-scale, geochemically-distinct provinces observed on the surface. Also, the size of the inner core (grey sphere at the center) is highly uncertain. These are just a few of the open issues that the upcoming ESA mission BepiColombo aims to address. (Image credit: N. Tosi, based on the simulations in Tosi et al. (2015), surface layer: NASA/Johns Hopkins University Applied Physics Laboratory/Carnegie Institution).
To first order, these observations can be accounted for by geodynamic models, which provide fundamental insights into the thermal and chemical evolution of Mercury’s interior (e.g., Tosi et al., 2013; Hauck et al., 2018; Fig. 1). Yet, major questions remain open. For example, the lack of a robust determination of the size of the inner core, together with uncertainties in its composition, prevents establishing conclusively the actual mechanism responsible for driving convection in Mercury’s liquid core and powering the present-day magnetic field. Furthermore, MESSENGER spectrometers delivered information on the distribution of major rock-forming elements of the northern hemisphere (due to the elliptical orbit, spectral measurements of the southern hemisphere lack sufficient resolution). Such a distribution implies the existence of various geochemically-distinct provinces (e.g., Weider et al., 2015). However, while the geochemical diversity associated with large impact basins – such as the 1500-km-wide Caloris – may be a consequence of the interaction between basin-forming impacts and the underlying mantle convection (Padovan et al., 2017), other large-scale features cannot be accounted for by existing models of Mercury’s interior.
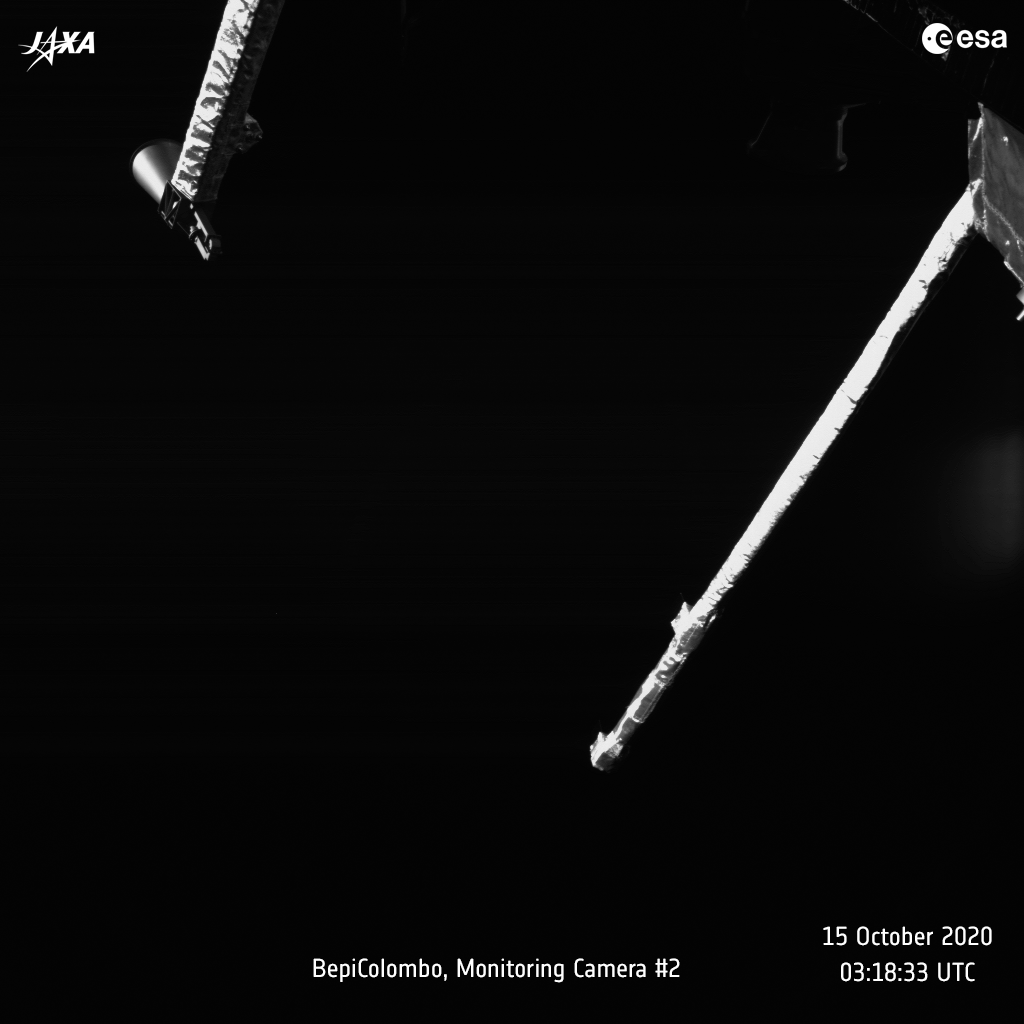
Fig. 2. BepiColombo had its closest approach to Venus during a flyby on October 15, 2020. Its monitoring cameras took this sequence of images showing Venus that appears on the right, behind the magnetometer boom (Credit: ESA/BepiColombo/MTM). Before entering Mercury’s orbit in December 2025, BepiColombo will perform another flyby of Venus and six flybys of Mercury.
After one flyby of Earth in April 2020, a second one of Venus in October (Fig. 2), and more to follow in the next few years, BepiColombo is scheduled to enter Mercury’s orbit in December 2025. With a launch mass of 4100 kg and the size of a small bus, BepiColombo is carrying two spacecrafts: the Mercury Magnetospheric Orbiter (MMO) and the Mercury Planetary Orbiter (MPO). While MMO will probe the exosphere and magnetosphere, MPO will fly closer to the surface, in an orbit that will allow probing in detail both the northern and the less well explored southern hemisphere with a suite of eleven instruments. Precise gravity and altimetry measurements will place a tight constraint on the size of the solid inner core (Steinbrügge et al., 2019) and provide much needed insight into the mechanism behind magnetic field generation and its relation to Mercury’s thermal history. MPO’s spectrometers will not only improve our knowledge of the distribution of major elements on the surface. For the first time, mineralogical mapping will be possible. Resolving the geochemical diversity of the entire surface at a uniform resolution will provide a key link to the composition of the interior and its dynamic evolution.
Further reading
- Byrne, P. K., Klimczak, C., Şengör, A. C., et al. (2014). Mercury’s global contraction much greater than earlier estimates. Nature Geosci., 7(4), 301.
- Byrne, P. K., Ostrach, L. R., Fassett, et al. (2016). Widespread effusive volcanism on Mercury likely ended by about 3.5 Ga. Geophys. Res. Lett., 43(14), 7408–7416.
- Hauck, S. A., Grott, M., Byrne, P. K., Denevi, B. W., Stanley, S. and McCoy, T. J. (2018). Mercury’s global evolution. In S. C. Solomon, L. R. Nittler and B. J. Anderson (Eds.), Mercury: The view after MESSENGER. Cambridge University Press.
- Head, J. W., Chapman, C. R., Strom, R. G., et al. (2011). Flood volcanism in the northern high latitudes of Mer- cury revealed by MESSENGER. Science, 333, 1853–1856.
- Padovan, S., Tosi, N., Plesa, A. C., and Ruedas, T. (2017). Impact-induced changes in source depth and volume of magmatism on Mercury and their observational signatures. Nature communications, 8(1), 1-10.
- Rivoldini, A., & Van Hoolst, T. (2013). The interior structure of Mercury constrained by the low-degree gravity field and the rotation of Mercury. Earth Planet. Sci. Lett., 377, 62–72.
- Solomon, S. C., Nittler, L. R. and Anderson, B.J. (Eds.) (2018). Mercury: The view after MESSENGER. Cambridge University Press.
- Tosi, N., Grott, M., Plesa, A. C., and Breuer, D. (2013). Thermochemical evolution of Mercury’s interior. Journal of Geophysical Research: Planets, 118(12), 2474-2487.
- Tosi, N., Čadek, O., Běhounková, M., Káňová, M., Plesa, A.‐C., Grott, M., Breuer, D., Padovan, S., Wieczorek, M.A. (2015). Mercury’s low‐degree geoid and topography controlled by insolation‐driven elastic deformation. Geophysical Research Letters, 42(18), 7327-7335.
- Weider, S. Z., Nittler, L. R., Starr, R. D., et al. (2015). Evidence for geochemical terranes on Mercury: Global mapping of major elements with MESSENGER’s X-Ray Spectrometer. Earth Planet. Sci. Lett., 416, 109–120.