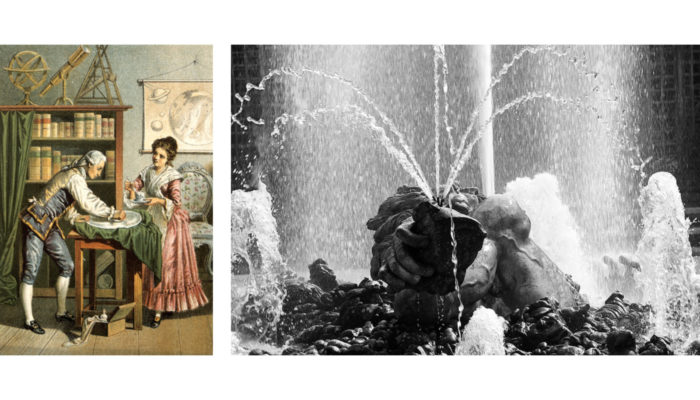
This week, Gael Choblet, CNRS research associate in the Laboratoire de Planétologie et Géodynamique (University of Nantes and Angers), tells us everything about the interior of Enceladus, an interesting icy moon of Saturn!
This is my first contribution in these pages. The choice of Saturn’s small moon Enceladus as a topic mostly results from my acquaintance with this planetary body. Yet, the reader probably remains to be convinced that this particular subject belongs to that specific section of EGU Geodynamics blog – I also do, although I noticed that some colleagues have proposed texts that are adjacent to the “geodynamics” central theme, albeit on far more important or at least far more urgent topics than Enceladus research. The ongoing extinction of living species is probably more pressing than the discovery of life outside the Earth, of which Enceladus could be one of the most accessible laboratories (probability not provided here – incidentally, this is not the subject of this text). Given this, the idea I propose in the following lines is to highlight the connection between progress in the knowledge on Enceladus’ interior and Geodynamics as a discipline, using a chronological approach. In the end, we (author, reader) will see whether this choice made sense: whether a dual perspective, exotic and familiar, ensues or whether the bridges built prove artificial and ephemeral.
Full disclosure: I have co-authored some of the work I mention and the following account certainly involves bias.
Enceladus, the moon, is known to humans since the middle of the french revolution although not because of it. Saturn being more distant than Jupiter, the discovery of its largest moon Titan waited 45 years after Galileo had spotted Jupiter’s four large moons of approximately the same size. In the case of Enceladus, almost precisely one order of magnitude smaller than Titan in radius, further instrumental developments (and a huge telescope, about one order of magnitude larger than Galileo’s) were necessary for german-born William Herschel, appointed Astronomer of the King of Great Britain at the time, to discover Enceladus. William’s younger sister Caroline probably helped: besides knowing how to make tea (illustration) and being also german-born, she participated in her brother’s astronomical research and conducted her own. For this reason, she won the Gold medal of the Royal Astronomical Society then became a member (first woman to achieve each of these distinctions).
Then, strangely enough, humankind lost interest in Enceladus.
Establishing a first connexion here with the Earth is hazardous since little is known by me about human’s discovery of its birth planet.
That Enceladus surface is made of water ice appeared likely in the 1970s, even before direct spectro- scopic evidence, owing to the bulk density of icy moons as well as the fact that Saturn’s rings spectra seem compatible with that of H2O ice. French engineers had already associated Enceladus to water when designing a fountain for the “Bosquet de l’Encelade” in the gardens of the Versailles castle (illus- tration). Yet, one has to admit that rather than a dazzling prescience (the moon was not known yet), their choice of water as a material was probably motivated by the inability to construct a magma foun- tain: Enceladus (the mythological figure), was one of these proto-gods, brothers and sisters of Saturn, whose access to fame mostly sums to their defeat against the (true) olympian deities. Early geodynam- icists often explained the known volcanic activity at the time by the fact these Giants/Titans were then buried beneath the ground and angry. For example, Giant Enceladus is told to lie beneath the ground of mount Etna after Athena gave him a beating. This volcanic character probably motivated the choice of a powerful water jet for the bosquet, which could also be fantasized as some form or foreknowledge, a concept not easily favored by geodynamicists.
So, water. Lots of water. Earth’s hydrosphere corresponds to a 2.5 km-thick shell at the surface. In fact, so little water that solid Earth’s topography is enough for continents to emerge and, strange thing (or not, you tell me), mountains are roughly as high as oceans are deep. Nothing of the sort for Enceladus: the hydrosphere thickness is 60 km (for a global radius of about 250 km) so that the surface of the rock component lies very deep beneath the surface. To say something slightly different in a slightly other way: water is half of Enceladus mass while the most extravagant hypotheses for Earth (if a number of water oceans are stored in the mantle and even more were present in the core) still provide a budget smaller than 2 %.
Quite early after I was born although not because of this, bold colleagues suggested that Enceladus’ location in the Saturn’s system, in the densest region of the otherwise very diffuse E-ring made of tiny ice particles, could be at the origin of this peculiar ring: as we will see below, this would prove a correct guess.
Because then occurred the real birth of Enceladus as a major body for planetary science (yes, knowledgeable reader, I intentionally mute the achievements of the Voyager spacecrafts for the sake of brevity): in the second half of 2004, the long planned Cassini-Huygens mission arrived in Saturn’s environment after a journey of about 7 years. Over the course of a remaining 13 years-long mission, this unique spacecraft with the mass of an elephant (launch mass: African bush elephant; dry mass: African forest elephant), carrying an impressive series of instruments developed in the 1990s fulfilled all the scientific expectations. Saturn itself and the architecture of the Saturn’s system were scrutinized. The planetary-size moon Titan (larger than Mercury) which, everything suggested, was certainly a fascinating body but whose orange glow formed by a dense atmosphere had removed the surface from the endeavors of previous space probes, turned out to be a fascinating body, arguably more so. For the reader more versed in serendipity, nevertheless, Enceladus’ activity is definitely the favorite pick in the list of Cassini’s top-ten discoveries. During the first months of Cassini’s Saturn trip, it occurred that a conductive cloud was located above Enceladus’ south pole. Further examinations by the multiple instruments revealed that this plume emerged from the moon (illustration), was composed of water (ice and vapor) and contaminants ejected from Enceladus interior through individual jets emanating from large parallel fractures in Enceladus’ icy crust (illustration) and, yes, Enceladus plume is feeding the E-ring (illustration). While Earth’s history of magmatism is known to significantly contribute to the evolution of its atmosphere, Enceladus’ gravity at the surface, a hundred times smaller, rather enables its volcanic activity to shape its orbital environment.
Still, such an activity remained puzzling given the moon’s dimension: infrared emission in the south polar region only (heat flowing through other terrains is too small to be detected) was soon estimated to be 10-15 GW. Equivalent to the geothermal power used by humans worldwide, this amount might seem modest to a non-geodynamicist as a negligible fraction of the Earth’s heat budget of internal origin (46 TW). Yet, the reader will have noticed that, given its size and rock content, Enceladus could be expected to expel only 50 times less energy if radiogenic decay were the sole provider of heat. This might not come as a surprise, though, for the planetary scientist aware of the peculiar heat budget of some icy moons: after all, Cassini’s older sibling devoted to the exploration of the Jupiter system, the Galileo spacecraft, had already estimated that 100 TW were transported through the volcanic surface of Io, the closest of the Galilean moons, and that the existence of Europa’s putative internal ocean also required another powerful heat source, identified even before the spacecraft observations to likely result from the dissipation of tidal deformation enabled by these two satellites’ orbits.
In the case of Enceladus, the localized emission at the South Pole, accompanied by complex tectonic features there confirming the ongoing activity witnessed by Cassini, led part of the research community to hypothesize the deep presence of a regional sea in the Southern hemisphere, beneath the ice, above the rocky core. Only such a liquid layer decoupling the motion of the ice shell and the rocky core would permit a sufficient deformation to dissipate large amounts of heat – viscous dissipation of tidal deformation was mostly envisioned in the ice layer as the supposedly cool rocks appeared too stiff, contrary to Io and its much hotter rocky mantle that most probably includes an asthenosphere much more developed than the Earth’s at present.
This was problematic, nevertheless. The poor knowledge of Saturn’s interior and possible dissipation mechanisms it may host implies that estimates of dissipation only rely on the observed motion of its natural satellites. At the time (second half of 2000s), this led to envision a necessarily episodic release of heat by Enceladus, in the fashion of Wilson cycles on the Earth where deviations of 25% around the long- term trend of the mean oceanic heat flux are postulated. Only, in the case of Enceladus, this episodic outburst appeared really exceptional (at best corresponding to maybe 1% of the duration of the moon’s duty cycle). A fairer comparison would thus be with the almost mythological catastrophic resurfacing event once postulated to explain the crater distribution on Venus if this one involved the recycling of a significant part of the lithosphere – a view that is probably in the process of being abandoned, anyway. At this stage of Enceladus research (early 2010s), the community was left with the difficult idea that it might be witnessing precisely an extraordinary phase of the evolution of the moon, a comforting notion possibly being that the sample of icy moons has a cardinal number larger than that of the terrestrial planets of the solar system (to the point where the use of statistics could almost begin to make sense).
Putting aside these uncertainties, a team composed Czech colleagues and people in our group in Nantes proposed an original way to constrain the interior of Enceladus. In the absence of seismology, a now classic approach for the study of planetary interiors relies on the use of the global shape (or topography) and gravity field, even if the results are more uncertain (more interior models satisfy a given observation). But in the case of Enceladus, a small moon, volcanic activity is so powerful that it could also reflect the global structure. Cassini has shown variations in the amplitude of this activity measured in terms of particle flux (the quantity of gas seems more or less constant) during the moon’s orbit. Never absent, it seems maximum at a precise point in the orbit (with a period of a little more than a day) which is repeated from one orbit to another. This seemed to indicate a control of the activity by tidal stresses that evolve with the position of Enceladus around Saturn and confirmed the prominent role played by tides. But this activity is delayed when compared to what would be expected of an elastic body: although other phenomena may contribute to this delay, we have bet that the viscous structure of the moon could be at the origin of it. We therefore built a method (certainly less precise than seismology, and with more uncertain foundations) to probe models of internal structure in terms of the rheological behavior that they oppose to tidal forcing. Several families of models could be suitable, some with a global ocean. But probably guided by a more or less conscious bias not unfamiliar to the geodynamicists that contributed to the debate on plate tectonics, our favorite candidates belonged to a group with a deep regional sea (illustration). Which proved wrong.
Unlike in the case of plate tectonics, the paradigm shift was very rapid. The causes of this effectiveness would still have to be studied but rather than a change in the nature of scientific practice, I imagine (without any proof) that this shall be attributed to a much smaller research community as well as to the speed of change of opinions since the Cassini spacecraft mowed down the models as fast as new radar swaths were acquired. In fact, about a year before the end of the mission (2017), the meticulous evaluation of the motion of Enceladus’ surface from the compilation of numerous Cassini images indicated a very marked dynamic of the moon’s rotation (precisely, its physical libration, the motion that enables the visible side of the Moon from the Earth to represent a little more than half of the total surface). Unequivocally, such an amplitude made it necessary that the ice shell was free to move without being mechanically anchored to the rocky core. As a french president said to describe a completely different subject, the idea of a regional sea had made “pschitt”.
With this powerful estimate of the libration as well as chemical evidence that jets emerged from a salty water reservoir, Enceladus became the planetary body for which the existence of a global internal ocean is the least doubtful (illustration). It is now emblematic of an increasingly large family named since “Ocean worlds”.
In the meantime,
- colleagues had demonstrated that dissipation in the Saturnian system could be much larger than anticipated so that the present-day activity of Enceladus might in the end correspond to a steady-state,
- two independent analyses of the jets materials and ice particles in the E ring (tiny sand grains and endogenic hydrogen) revealed the likelihood of ongoing hydrothermal activity, pushing Enceladus in the short list of planetary bodies worth studying for the emergence of life (all the more so that the ocean delivers samples to spacecrafts flying through the plumes (as did Cassini several times) or would land on the surface, beneath the snow).
But this takes us away from geodynamics.
In the second and last episode, dear reader, I will show
- how the principle of isostasy dear to geodynamicists since the 19th century was key to finally (?) understanding the interior of Enceladus,
- how the thermal convection dear to geodynamicists from the beginning of the 20th century to describe the Earth’s mantle might also occur within the rocky core of Enceladus, with different modalities,
- and perhaps how oceanic hotspots dear to geodynamicists from the second half of the 20th century might bear resemblance to Enceladus’ erupting centers (or not).
Join us next time, won’t you?