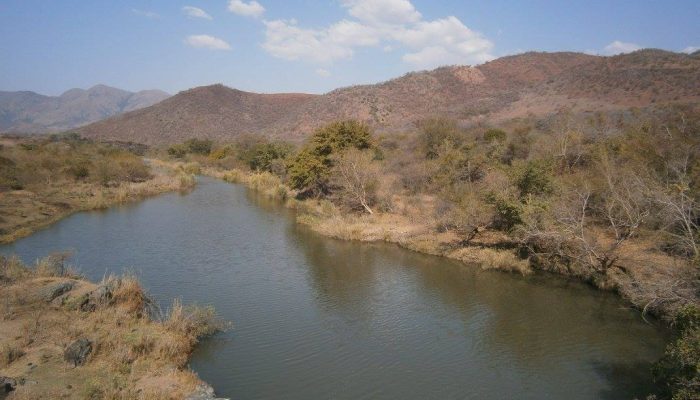
The earliest continents played a fundamental role on Earth’s habitability. However, their generation is still not understood, and it requires an integrated approach between petrology and geodynamic modelling. In a new study, Piccolo and co-workers developed a method to handle the effects of chemical evolution on the geodynamic processes. They show that the production of the earliest felsic crust triggers a self-feeding chain of events that leads to the generation of the first proto-continents.
The Archean Eon (4.0-2.5) is the first act of the evolution of life and represent one of the first steps of Earth towards its present-state. Only few remnants have reached us representing different space-time windows that are difficult to reconcile in a unique interpretative framework. Many questions are still unsolved, and all the answers may be interconnected. One of them is related to the generation of the continental crust, which played a fundamental role on the developing of the biosphere as we know now. Although the present-day sites of production are all most understood, we still try to grasp the dominant process that were generating its constituents (e.g. granitoids) during the Archean. Herein the main “hot” topic is the felsic components of the continental crust, (i.e. felsic crust/melts), and their generation during the Archean, following the recent results from Piccolo et al. (2019) [1].
Felsic melts cannot be directly produced from a partially molten mantle source; therefore, silica-rich magma generation must occur via a multistage process [2] that comprises at least two main steps: i) extraction of raw mafic materials; ii) differentiation via fractional crystallization or via partial melting of the hydrated basalts. Both processes come at costs, and, in fact the generation of felsic melts implies the production of large volume of complementary mafic/ultramafic dense residuum (the solid fraction of a partially melted rock), which is not observed in geological records. Such residual rocks have a different chemical composition with respect to the parental magma, being more mafic, and thus potentially producing denser minerals. During the Archean the most widely accepted recipe to produce felsic crust is to bake hydrated meta-basalts at high pressure until they partially melt [3]–[5].
Available Archean geological records are not enough to provide a coherent picture. Therefore, it is natural to assist the interpretation of available data with indirect means such as numerical and petrological modelling. Moreover, it is necessary to employ an integrated approach between geodynamic and petrology to study the effect of the chemical evolution on the overall dynamics of the lithosphere. In Piccolo et al. (2019), the authors accepted this challenge by conjugating petrological phase diagrams with geodynamic simulations to handle a simple — but petrologically robust — chemical evolution of the mafic protolith. They produce a set of genetically linked phase diagrams for both mantle and mafic crustal compositions — exploiting the recent advances on thermodynamic modelling for mafic/ultramafic systems (for further details, [5]–[8]) — to simulate the chemical evolution as a function of the melt extracted. Then, they modify their finite element code (MVEP2) to change the compositional phase as a function of the principal mineralogical transformation.
The base line scenario comprise a lithosphere (composed by hydrated effusive basalts, intrusive rocks and a residual lithospheric mantle) underlaid by a fertile asthenosphere featuring high temperature and radiogenic heat production consistently with Archean conditions [9], [10]. During the initial stage of the experiments, asthenosphere self-heats above solidus, resulting in the genesis of more mafic melts that are readily extracted and converted into mafic hydrated basalts and dry intrusions. The latter are emplaced within the lower crust and heat the overlying hydrated units that start to partially melt. Such two-steps processes have two main consequences: the production of the first granitoids that are emplaced at middle-crust depths and the production of large amount of dense mafic residuum. Such rocks are not so common in the geological record, as stated above. Therefore, the inevitable question is: what are the processes that assists their disposal? The solution of this conundrum relies on the density contrast between the underlying mantle and complementary mafic residuum. If the density contrast is sufficient high, any geometrical and thermal perturbation between them can trigger Rayleigh-Taylor drip instabilities. The foundering of such drip into the mantle forces the weak asthenosphere to upwell causing more mafic melts and thus inducing more felsic crust production and consequent gravitational instabilities. After a few million years the mantle is quiescent because its temperature significantly drops because it mixes with the dripped mafic while the crust start being more enriched of felsic crustal components. Several parameters have been tested, and the main chains of events have been replicated in a plethora of conditions, especially at lower mantle temperature, suggesting that the generation of felsic crust is associated to mantle cooling events, that may buffer the upper mantle temperature.
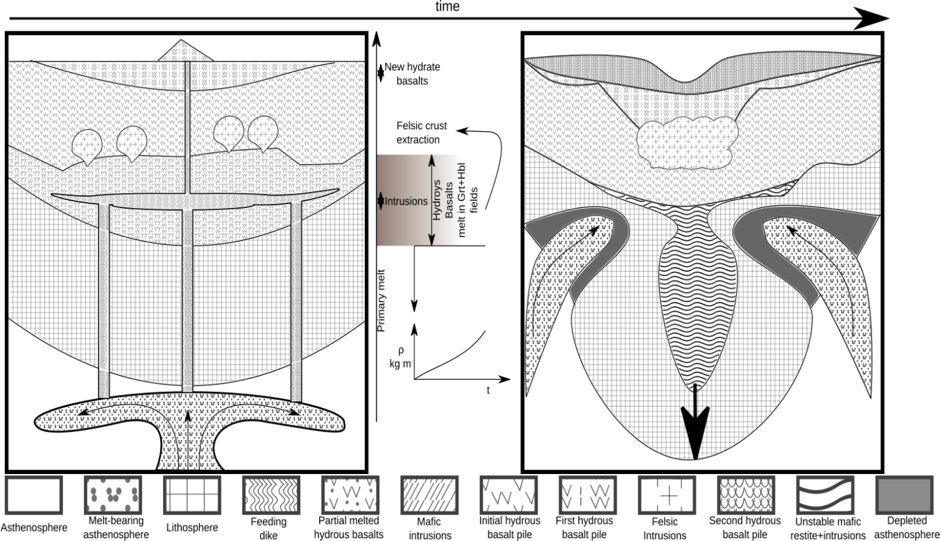
Melt coming from the astenosphere percolates through the lithosphere, heating and both plastically and thermally weakening it. Part of the mafic melts are emplaced in the lower crust, while the remnants are erupted. As soon as a critical amount of residuum is reached, RTIs are triggered, and asthenosphere fills the space left by the delaminated lithosphere. This results in a feedback between mantle melting and new felsic crust production. From ref. [1].
The removal of the residuum implies production of more mafic melts, and thus more felsic crust that leads inevitably to again to gravitational instabilities yielding a self-feeding. Moreover, any vertical tectonic setting fuelled by mantle magmatic processes is suitable to produce proto-continents thank to these feedbacks. Such findings are consistent with many independent line of research (e.g., [11], [12], [13]) and the novelty of this study relies in a first attempt to conjugate realistic petrological constraint with the chemical evolution induced by melt extraction.
References: 1. A. Piccolo, R. M. Palin, B. J. P. Kaus, and R. W. White, “Generation of Earth’s early continents from a relatively cool Archean mantle,” Geochemistry, Geophys. Geosystems, 2019. 2. R. L. Rudnick and S. Gao, “4.1 – Composition of the Continental Crust,” in Treatise on Geochemistry, 2014, pp. 1–51. 3. J.-F. Moyen and G. Stevens, “Experimental constraints on TTG petrogenesis: implications for Archean geodynamics,” Archean Geodyn. Environ., pp. 149–175, 2006. 4. R. P. Rapp, N. Shimizu, and M. D. Norman, “Growth of early continental crust by partial melting of eclogite,” Nature, vol. 425, pp. 605–609, Oct. 2003. 5. R. M. Palin, R. W. White, and E. C. R. Green, “Partial melting of metabasic rocks and the generation of tonalitic???trondhjemitic???granodioritic (TTG) crust in the Archaean: Constraints from phase equilibrium modelling,” Precambrian Res., vol. 287, pp. 73–90, 2016. 6. R. M. Palin, R. W. White, E. C. R. Green, J. F. A. Diener, R. Powell, and T. J. B. Holland, “High-grade metamorphism and partial melting of basic and intermediate rocks,” J. Metamorph. Geol., vol. 34, no. 9, pp. 871–892, 2016. 7. E. C. R. Green, R. W. White, J. F. A. Diener, R. Powell, T. J. B. Holland, and R. M. Palin, “Activity–composition relations for the calculation of partial melting equilibria in metabasic rocks,” J. Metamorph. Geol., vol. 34, no. 9, pp. 845–869, 2016. 8. R. W. White, R. M. Palin, and E. C. R. Green, “High-grade metamorphism and partial melting in Archean composite grey gneiss complexes,” J. Metamorph. Geol., vol. 35, no. 2, pp. 181–195, 2017. 9. C. T. Herzberg, K. C. Condie, and J. Korenaga, “Thermal history of the Earth and its petrological expression,” Earth Planet. Sci. Lett., vol. 292, no. 1–2, pp. 79–88, 2010. 10. J. Ganne and X. Feng, “Primary magmas and mantle temperatures through time,” Geochemistry, Geophys. Geosystems, vol. 18, no. 3, pp. 872–888, Feb. 2017. 11. J. H. Bédard, “A catalytic delamination-driven model for coupled genesis of Archaean crust and sub-continental lithospheric mantle,” Geochim. Cosmochim. Acta, vol. 70, no. 5, pp. 1188–1214, 2006. 12. T. E. Zegers and P. E. van Keken, “Middle Archean continent formation by crustal delamination,” Geology, vol. 29, no. 12, pp. 1083–1086, 2001. 13. E. Sizova, T. V. Gerya, K. Stüwe, and M. Brown, “Generation of felsic crust in the Archean: A geodynamic modeling perspective,” Precambrian Res., vol. 271, pp. 198–224, 2015.