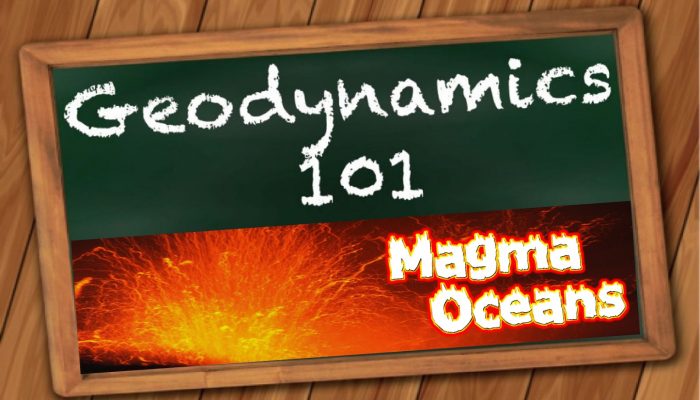
The Geodynamics 101 series serves to showcase the diversity of research topics and methods in the geodynamics community in an understandable manner. We welcome all researchers – PhD students to professors – to introduce their area of expertise in a lighthearted, entertaining manner and touch upon some of the outstanding questions and problems related to their fields. For our latest ‘Geodynamics 101’ post we will talk about magma oceans!
When you think about the Earth a long, long time ago, in its late stages of formation, which picture comes to your mind? A lot of volcanoes erupting? Lava running from cracks in rocks? Meteorites falling? Maybe dinosaurs roaming the land? Well, first of all, there was certainly no life, and the dinosaurs only showed up somewhat recently in the history of the Earth (~240 million years ago, while the Earth is ~4500 million years old). In fact, our planet was a much more extreme environment that one might think… Even if frequent, meteorites would only arrive every few thousands of years. Temperatures at the surface were extremely high, as high as 2000 K (1727 °C). Yeah, forget it, too hot even to go to the beach. That, and… there was no land, because a big part of the rocks that form the Earth were molten and our planet was covered in a deep magma ocean. An ocean you definitely don’t want to go in for a swim.
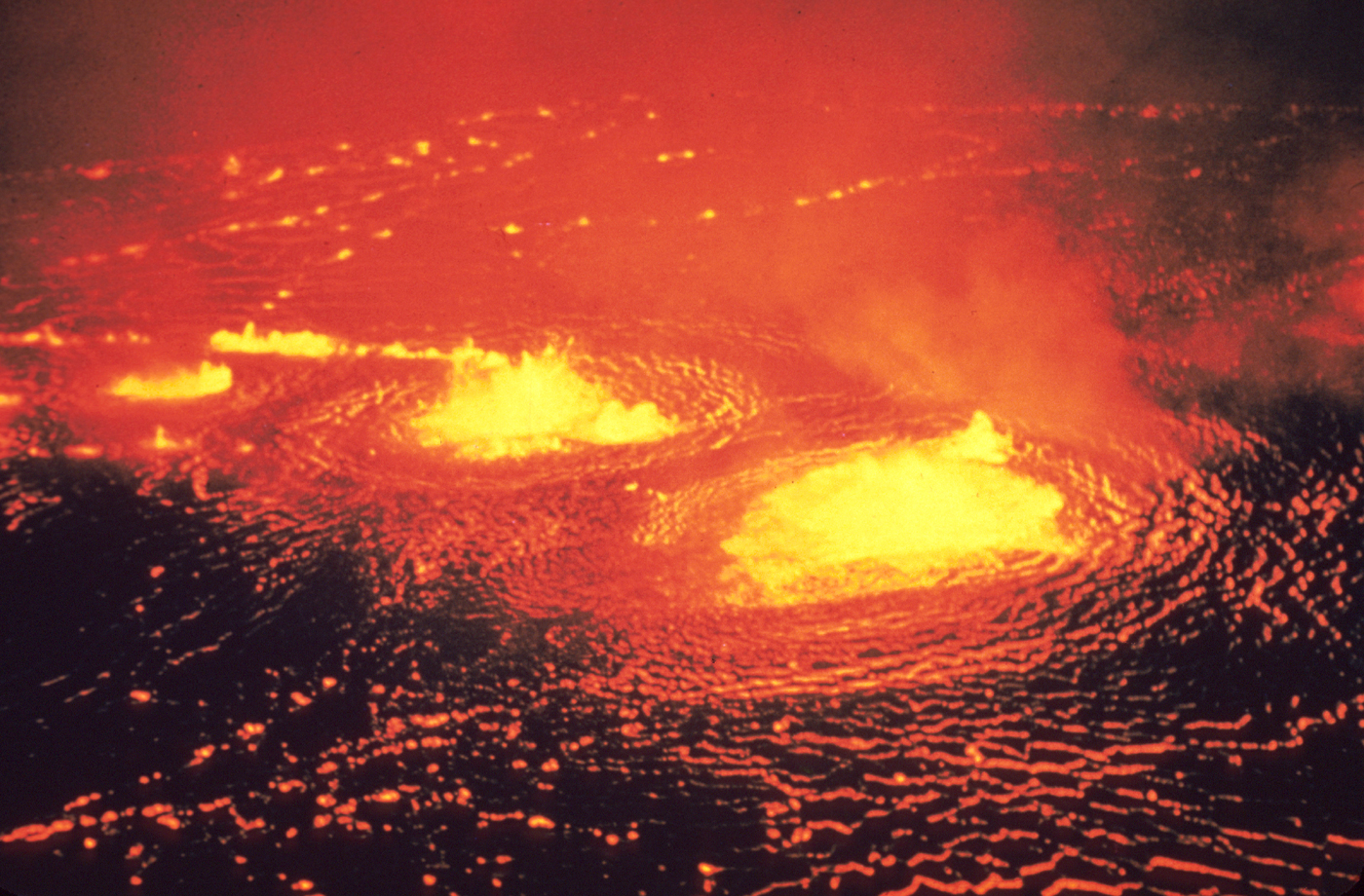
Weather prediction for the Earth, 4.5 billion years ago: really hot, the sort of hot that doesn’t allow you to work on your tan because… well, everything was molten. This photo was in fact taken in the Hawaii Volcanoes National Park, in May 1954 during the eruption of the Kilauea Volcano. Credit: photo by J. P. Eaton, May 31, 1954
What?! A magma ocean?
That’s right, a magma ocean. Think of it as a body of magma, consisting of a mixture of molten or partially-molten rock, volatiles (dissolved gas and gas bubbles) and solids (suspended crystals). The magma behaves as a liquid, which means that the quantity of solids floating in the system is not enough for the solid particles to connect with each other. When that happens, we are left with a crystal mush, where the magma flows inside a porous rock, instead of having solid particles floating in the liquid, as happens in a magma ocean.
A magma ocean is an extreme environment that is vigorously convecting. Several laboratory experiments suggest that the consistency of such systems is something like honey, with uncertainties depending on several factors such as pressure, temperature, the amount of water and the presence of crystals. Yeah, extremely hot molten rocks flowing like honey covering all the surface of the Earth and extending to thousands of kilometres depth. Very, very different from today’s Earth, hum? By definition a magma ocean needs to encompass a substantial fraction of the planet (something like more than 10%), otherwise we can call it a magma pond or a magma lake. No swimming in these as well. Also, no ducks there.
Sounds a bit like science fiction, right?
Yeah… However, the idea has been around for some centuries. In the late XVII century, Gottfried Wilhelm Leibniz proposed that Earth began as a uniform liquid, and differentiated compositionally as it cooled. Others, such as Comte de Buffon, or Lord Kelvin tried to calculate the age of Earth based on the assumption that the Earth was once completely molten and cooled to present-day (and is still cooling).
Ironically, humankind had to go to the Moon in order for the magma ocean hypothesis to evolve to its modern form. Samples returned from the Apollo missions showed that the whiter part of the crust you can see when you look up to the Moon is composed of rocks called anorthosites. In short, and without going into detail, the only mechanism for the formation of these rocks that has attained general acceptance is if they formed through flotation of crystals to the top of a magma ocean on the early Moon. Other rocks recovered from the Moon are also consistent with a fractional solidification of a magma ocean.
If the Moon went through a magma ocean stage it is likely that the Earth underwent it too. Just as the other rocky planets in the inner Solar System: Mercury, Venus and Mars. In fact, various observations point to the occurrence of magma oceans in the early evolution of rocky planets. We know this through the observation of, for example, iron meteorites, or on Earth because we know that a large iron core exists. A magma ocean facilitates the formation of the core early in the history of the planets. Iron is a heavy metal that is thought to have arrived on the early Earth mixed with silicon and silicates. A very hot magma ocean would have melted the rocks and metals and allowed the heavier liquid iron to sink down to the centre of the Earth to form the core.
Thus, it is widely accepted that magma oceans are significant events in the earliest stages of planetary evolution and set the initial conditions for their future evolution. Meaning that understanding how a magma ocean evolved on Earth is key to understand why life exists on our planet.
How did magma oceans form?
The late stage of formation of the Earth and other rocky planets was an energetic process, where violent impacts between protoplanets happened. The energy provided by impacts was the largest energy source to heat and melt the Earth. There were however other sources of heat I should mention such as the conversion of gravitational energy of formation into heat, heat losses from the core at the core-mantle boundary, radioactive decay (mainly short-lived isotopes such as 26Al and 60Fe, which are important in the early stages of planetary formation), electromagnetic induction heating and tidal heating (caused by the proximity to another large object). Basically, a large amount of energy was being provided to the juvenile rocky planets. Thus, again, it is very likely they underwent one or multiple large-scale melting events.
It is probable that in the last giant impact to hit the Earth, the impactor was the size of Mars. Such a big impact had enough energy to melt at least a substantial part of the mantle. Oh, and this impact is also believed to have formed the Moon. Pretty cool, right? The Moon-forming impact was most likely in the origin of the last major, deep and global magma ocean on Earth. But how did the magma ocean evolve in such a way that set up the initial conditions for the evolution of a tectonically active planet, able to sustain water at the surface, an atmosphere, and life?
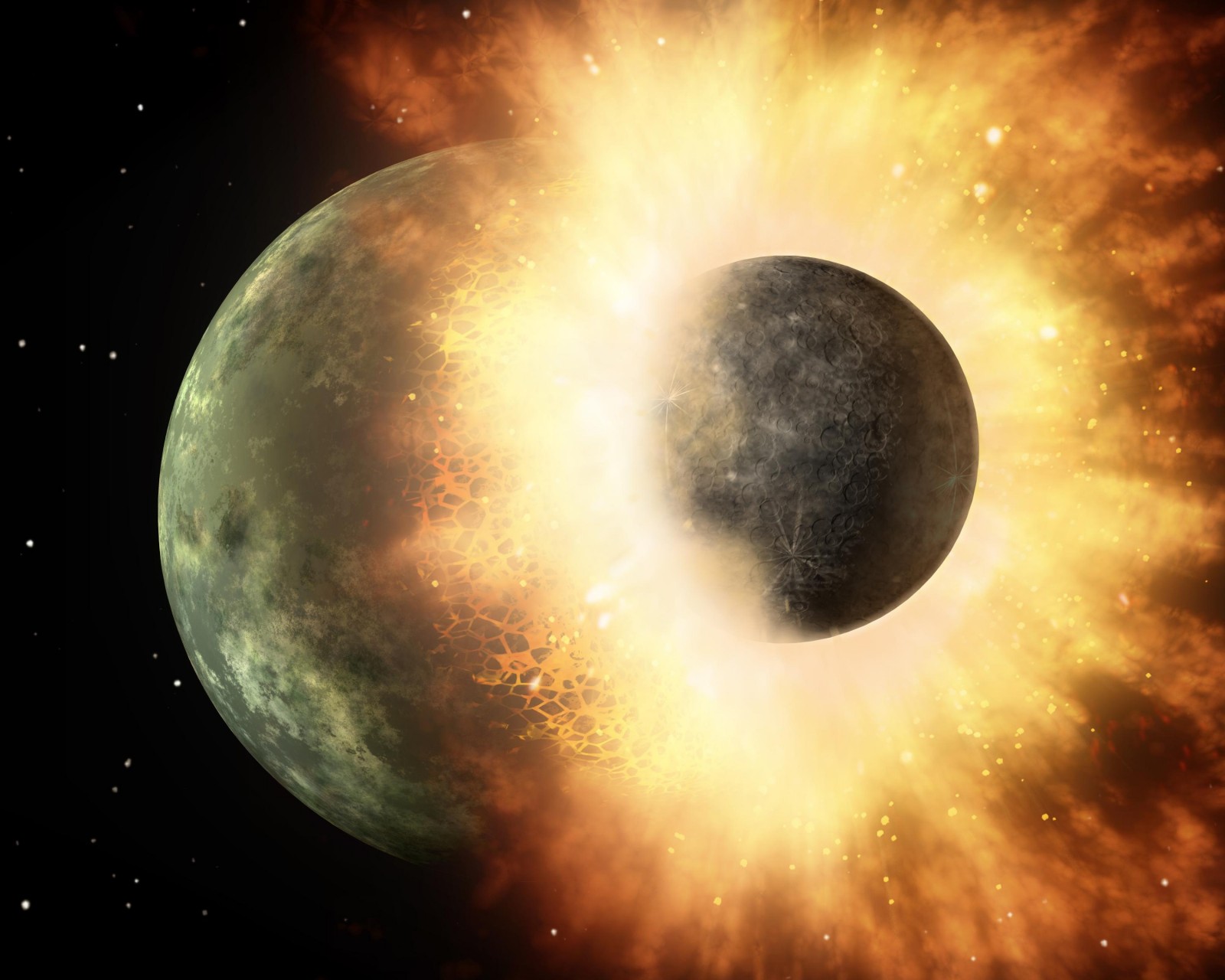
BOOOMMM! And the Earth was molten! And the Moon was formed! Artist’s depiction of a collision between two planetary bodies. Such an impact between Earth and a Mars-sized object likely formed the Moon. Credit: NASA/JPL-Caltech
How did the magma ocean evolve on Earth?
Fortunately for us, the magma ocean solidified, and Earth’s conditions are very different from back then. According to the conventional view, as a magma ocean loses heat from the surface, its temperature drops, and it starts crystalizing from the bottom-up. When there are enough crystals present, a solid matrix is formed, and because overall this structure flows slower, the heat loss is also much slower, and thus crystallization is prolonged. Estimates for the transition from a liquid- to a solid-dominated regime range from thousands of years to several hundred million years. However, fully crystallizing the mantle takes billions of years. It might sound a bit strange that the uncertainty in the lifetime of a magma ocean exhibits such large differences. This is due to the fact that we don’t know much about such a system (we never observed one…), and the data available is rare and hard to interpret. These are the sort of uncertainties that we have to deal with when studying the evolution of a magma ocean on Earth:
- Was an atmosphere present? After the Moon-forming impact the surface temperatures were very high (~2000 K), so the heat transfer from the interior to the surface of the magma ocean is expected to be very rapid. But, these values can be buffered, and timescales of crystallization extended if an atmosphere is present. In the beginning an atmosphere composed of vaporized silicates (literally vaporized rock!) covered the Earth. Afterwards, as the magma ocean cools down, it degasses through bubbles that rise up and burst at the surface to form a thick and insulating steam-dominated atmosphere. Such atmosphere can substantially extend the cooling time by millions of years. As we will see next, this has important implications in the mode of crystallization of a magma ocean. Finally, once sufficient cooling is attained, the atmosphere collapses into a water ocean, and our planet starts to look more like the “Blue Planet” we recognize today.
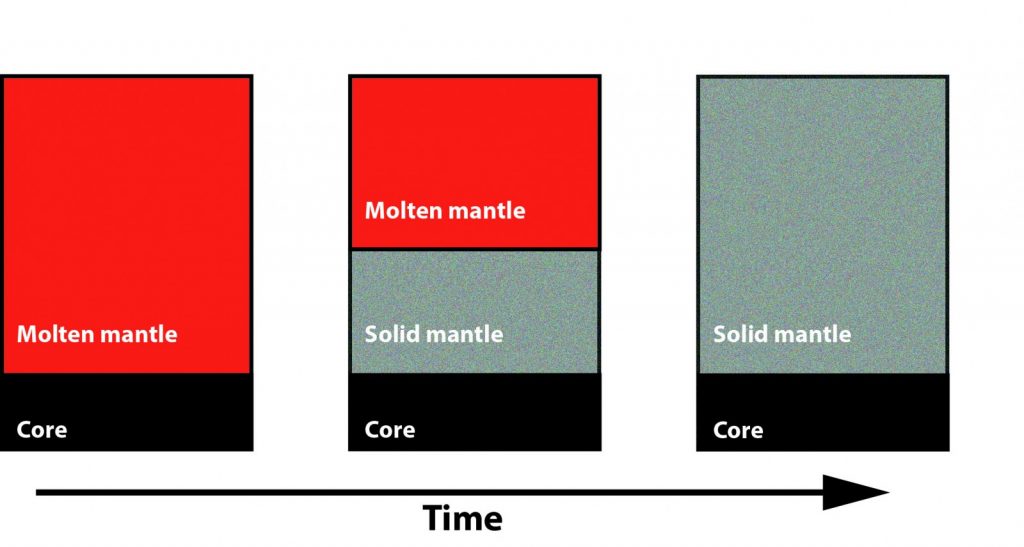
How does the magma ocean crystallize? In the classic view, shown here, it crystallizes from the bottom-up. Credit: Diogo Lourenço
- Could the surface be solid? The existence of a solid lid at the surface would slow down the cooling of a magma ocean, and therefore increase its solidification time. However, it is unlikely that such a lid developed in bigger planets because of: (1) the possible existence of an insulating atmosphere that keeps the temperature at the surface high, (2) the major possibility of any solidified material to sink, and (3) any small impactor during the cooling of the magma ocean would disrupt the formed lid. The only likely way to form a relatively complete conductive lid on a planetary-scale magma ocean is by flotation of buoyant minerals to the surface. Like in the Moon, remember? However, this mechanism is likely to occur only on small planets.
- How do crystals in a magma ocean behave? One of the most important and complex questions regarding the crystallisation of a magma ocean is whether the crystals continue to be suspended in the liquid, or whether they settle, being removed from the liquid magma ocean. This is a challenging problem to address due to the lack of information about the conditions in a magma ocean, and the complexity of the physics of settling versus entrainment in a vigorous fluid flow. In general, longer timescales of magma ocean freezing imply relatively slow convection within the magma ocean and may allow for crystal settling. On the other hand, short timescales imply fast turbulent convection, and hence no crystal settling. On Earth, with all the uncertainties, both styles are acceptable to have operated, but most likely a combination of the two types of solidification existed in a magma ocean, where at first crystals remained entrained, and as the magma ocean cools down crystals started to settle.
- Full-mantle overturns? Another complexity in the evolution of a magma ocean is that full-mantle overturns are predicted. Two types have been proposed: (1) Thermal overturns, where the (partially-molten) mantle resulting from the solidification of a magma ocean is gravitationally unstable. These instabilities can grow to a solid-state overturning of the cumulate mantle. (2) “Compositional” overturns. As the magma ocean crystallizes, a progressive enrichment of iron and incompatible elements in the magma is expected, and ultimately, this leads to an unstable compositional density stratification because magma ocean cumulates will be denser as the crystallisation front proceeds. A single overturn and a pronounced stratification of the compositionally stratified cumulate layers are expected, which could delay mantle solid convection for billions of years. However, it might be the case that stratification is (partially) erased through progressive mixing due to multiple incremental cumulate overturns, instead of a single one.
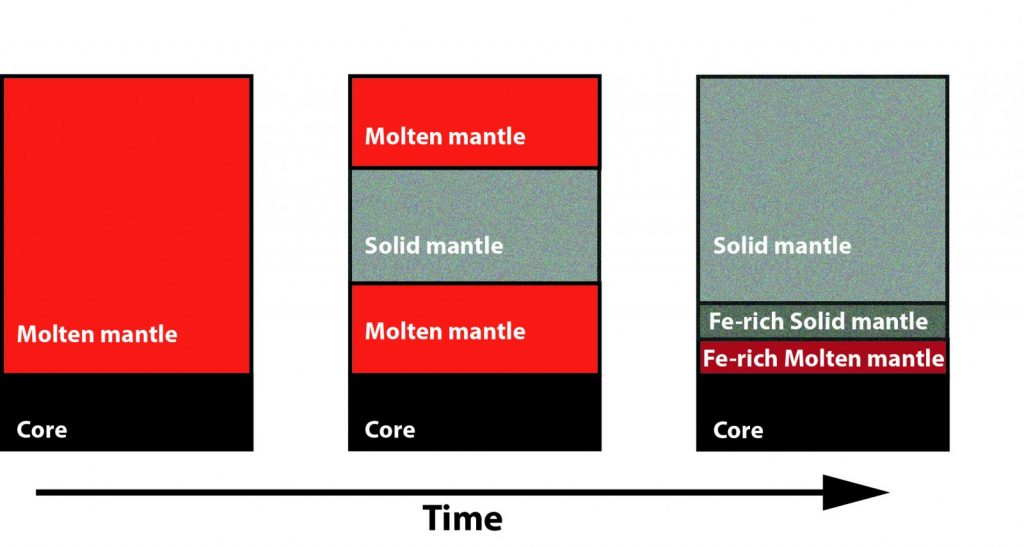
How does the magma ocean crystallize? In a more more recent view, shown here, it crystallizes from the middle-out. Iron (Fe) tends to concentrate in the magma. Eventually, when the BMO is almost solid, the last-forming solids are highly iron-enriched, and probably dense enough to become stable against entrainment in the solid mantle. Credit: Diogo Lourenço based on Labrosse et al. (2007)
- Did the magma ocean really crystallize from the bottom to the top? Alright, here we go for another strange bit. The conventional view presented above is that the magma ocean crystallised from the bottom-up. Makes sense, yes? However, evolution from a completely molten state resulting from the Moon-forming impact might have been different from the conventional view. Recent measurements suggest that it is possible that liquids are denser than solids at lowermost mantle depths, opening the possibility for alternative scenarios of crystallisation of a magma ocean from mid-mantle depths. This would imply that as the magma ocean crystallizes, a shallow and a basal magma ocean (BMO) are formed. The solid layer grows upward and downward, however it grows faster towards the surface because of rapid heat loss to the atmosphere. The BMO’s heat loss is buffered by the mantle, so it crystallizes much slower. Moreover, the basal melt layer is also cooling slower because iron and incompatible trace elements (such as heat-producing elements) would tend to be concentrated in the magma. Eventually, the BMO would almost completely solidify, with the last-forming solids being highly iron-enriched, and probably dense enough to become stable against complete entrainment in the solid mantle. These dense and solid piles could account for some of the observed large-scale features with reduced seismic velocities around the core-mantle boundary we observe today. Understanding whether the Earth had a BMO, and if so, its evolution, is a first-order question that has important implications for planetary thermochemical evolution, for example some reservoirs isolated from the rest of the mantle can be the result from the crystallisation of a BMO. Also, it would have affected the thermal history of the Earth and affected the history of the geodynamo in our planet.
Answering these questions, which are all connected to the chemical and dynamical evolution of a magma ocean, is very important in order to understand the initial conditions for solid-state mantle convection, which on Earth led to plate tectonics and life (but did not in other terrestrial planets). We should keep in mind that magma oceans are common and are still being formed in other galaxies, so maybe planets with magma oceans will one day become targets for direct imaging. Who knows?
I will leave you with some links and further reading below if you really got excited with this text! If you really really got motivated then you can also read my PhD thesis, I’m still recovering from that time, so it will make me happy to know it taught something to other people.
Alright, enough! Hope you enjoyed the read and remember to not swim in magma oceans (you might get arrested)! Bye-bye!
References and further reading: Abe, Y. (1997). Thermal and chemical evolution of the terrestrial magma ocean. Physics of the Earth and Planetary Interiors, 100:27-39. Ballmer, M. D., Lourenço, D. L., Hirose, K., Caracas, R., and Nomura, R. (2017). Reconciling magma-ocean crystallization models with the present-day structure of the earth’s mantle. Geochemistry, Geophysics, Geosystems, 18(7):2785–2806. Elkins-Tanton, L. T. (2012). Magma Oceans in the Inner Solar System. Annual Review of Earth and Planetary Sciences, 40(1):113–139. Labrosse, S., Hernlund, J. W., and Coltice, N. (2007). A crystallizing dense magma ocean at the base of the Earth’s mantle. Nature, 450(7171):866–869. Labrosse, S., Hernlund, J. W., and Hirose, K. (2015). Fractional Melting and Freezing in the Deep Mantle and Implications for the Formation of a Basal Magma Ocean. In The Early Earth Accretion and Differentiation, edited by Badro, J. and Walter, M., Hoboken, NJ. Lourenço, D. L. (2017). The influence of melting on the thermo-chemical evolution of rocky planets’ interiors. PhD thesis, ETH Zurich. [link] Rubie, D. C., Nimmo, F., and Melosh, H. J. (2007). Formation of Earth’s Core, In Treatise on Geophysics, edited by Gerald Schubert, Elsevier, Amsterdam, pages 51-90. Solomatov, V. S. (2007). Magma Oceans and Primordial Mantle Differentiation, In Treatise on Geophysics, edited by Gerald Schubert, Elsevier, Amsterdam, pages 91-119.