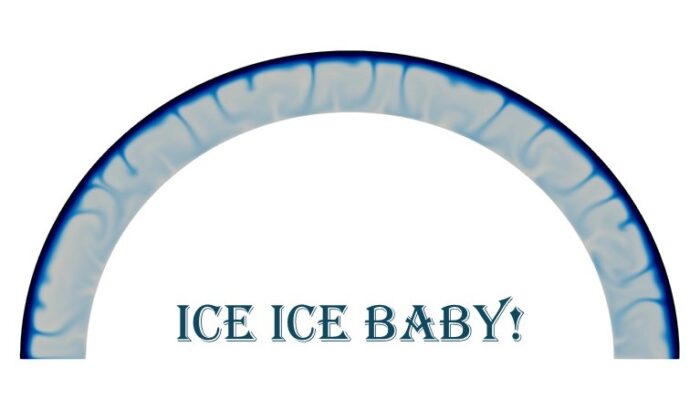
Ganymede, one of the Galilean moons of Jupiter and the largest in the Solar System, has caught the scientists’ attention due to its potential for hosting life. The JUICE mission, launched from the Guiana Space Centre in French Guiana on 14 April 2023, is on its way to orbit and conduct experiments on the Galilean moons (Ganymede, Europa, and Callisto), with particular emphasis on characterizing Ganymede’s internal dynamics, surface processes, and atmospheric conditions. In this week’s blog, Dr. Katherine Villavicencio (University G. d’Annunzio, Italy), writes about modelling the thermal evolution and the passive melt production within the outer ice shell of Ganymede.
My PhD thesis was focused on performing numerical geodynamic simulations of the thermal evolution within the outer ice shell of Ganymede. The purpose of these simulations was to investigate which are the parameters that might provoke melting within the ice layer and could be associated to surface processes such as cryovolcanism, polar deposits, and impact craters. Ganymede has a radius of 2630 kilometers, it is bigger than Mercury (2439 kilometers) and Pluto (1188 kilometers). It is the only moon in the Solar System with its own magnetic field, it orbits Jupiter at a distance of 1,070,000 kilometers, being the third one orbiting around the big gaseous planet before Io and Europa, and after Calisto. The internal structure of Ganymede is known as sandwiched, because it possesses a well differentiated layers starting from the very deep interior with an iron core, surrounded by a rocky mantle, followed by a high-pressure ice mantle, underlying a saline ocean layer, to finally a non-well constrained outer ice crust (figure 2).
Figure 2. Internal structure of Ganymede. The outer ice crust with a thickness ranging between 10 km to 150 km. A salty layer underlaying the ice crust and the HP mantle. Below there is a rocky mantle and in the innermost part it possesses an iron core. Photo taken from Collegedunia.com
In order to investigate how it can be possible to produce melt within the outer ice shell, it was performed a sensitivity analysis of the thermal evolution for compressible and incompressible fluids with strongly viscosity-temperature dependence using the open source ASPECT (Advanced Solver for Planetary Evolution, Convection, and Tectonics). By varying the reference viscosity (1013 , 1014 , 1015 Pa s), ice shell thickness from 10 km to 150 km, creep deformation, and pressure at the melting point of ice, we simulated the thermal evolution applying different compressibility approximations: for incompressible models, the Boussinesq Approximation (BA), and for compressible models the Anelastic Liquid Approximation (ALA) or the Isentropic Compression Approximation (ICA).
Among the mathematical equations that describe the physical processes, it was included the vigor of the convection given by the Rayleigh number, the strain rate based on creep deformation for ices, the viscosity-temperature dependence for Newtonian rheologies, the volumetric dissipation rate used for an incompressible Maxwell body, and the local production of melt computed by calculating the amount of heat available for melting which depends on the heat capacity and the latent heat. By computing all these equations, we aimed to answer the following questions such as: Does hydrated salty material relate to a cryovolcanic tectonic activity?, can the internal geodynamic activity influence on the deformation on icy surfaces?, does the presence of ammonia reduce the melting temperature and provoke a passive production of melt within the outer ice shell of Ganymede?
Figure 3. Anelastic experiments within a cartesian geometry with an ice shell thickness of 50 km, for models with composite viscosity. The reference viscosity is 1014 Pa s. The thermal evolution shows convective cells with thin and cold downwellings reaching the bottom of the models and warmer upwellings ascending until the bottom of the stagnant lid regime (up) at the top of both experiments. Pockets of molten material are ascending into the upwelling melted plume (down).
The snapshots from these simulations in figure 3 show that it is possible to produce melt within the outer ice shell of Ganymede under a restricted geophysical parameters using the Anelastic Liquid Approximation (ALA). The thermal patterns of the plumes (down) reveal pockets of molten material ascending throughout the upwellings and being more concentrated at the bottom of the models due to interaction with the ocean shell. These results will support the objectives of the RIME instrument on board of JUICE mission. They might help to have a better understanding of the internal mechanisms conducting heat on surface, leading to changes in and the geological and atmospheric processes. These models could also be used to predict how much energy transfer takes place at the limit between the subsurface ocean and the bottom of the ice shell. In future, there will be explored the effects of melt migration on surface, including an analysis of faults along cryovolcanism regions.
References. [1] Bangerth, W., Dannberg, J., Fraters, M., Gassmoeller, R., Glerum, A., Heister, T., et al. (2022). “ASPECT: Advanced Solver for Problems in Earth's ConvecTion, User Manual.” doi.org/10.6084/M9.FIGSHARE.4865333 [2] Choblet. et al., (2017). Heat transport in the high-pressure ice mantle of large icy moons and the dissipation number. Icarus 285, 252–26 [3] Jia et al., (2021). The magnetosphere of Ganymede. Magnetosphere S S. 557 – 573 [4] Schubert, G., Stevenson, D.J., Ellsworth, K. (1981). Internal structures of the Galilean satellites. Icarus 47, 46–59 [5] Schubert, G., et al., (2010) Evolution of icy satellites. Space Sci Rev 153:447–484 [6] Solomatov, V. S. (1995). Scaling of temperature- and stress-dependent viscosity convection, Phys. Fluids, 7, 266– 274.