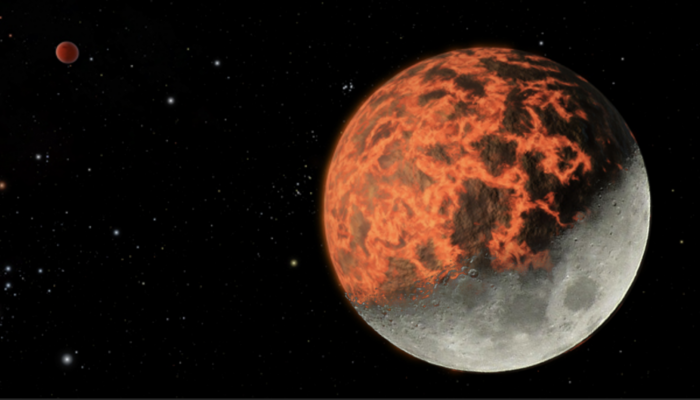
Geochemists and geophysicists, even with their favorite tools, have often scratched their heads while probing Earth’s deep interior—one can only imagine the challenges while applying those techniques to the Moon! This week in News & Views, Arkadeep Roy, a PhD candidate from the University of Arizona demonstrates how the intersection of experimental petrology, geochemistry, and geophysical modeling can uncover the selenotherm i.e., just how hot our closest neighbor’s interior is.
The Moon formed billions of years ago following a massive collision between the early Earth and another Mars-sized planetary body called Theia. The debris created due to the collision, consequently accreted to form the Moon. The accretionary process released an enormous amount of energy, leaving much of the Moon in a molten state, creating what scientists refer to as a “magma ocean”. Over time, the Moon gradually cooled which led to the crystallization of solid minerals which formed distinct layers like the crust and mantle.
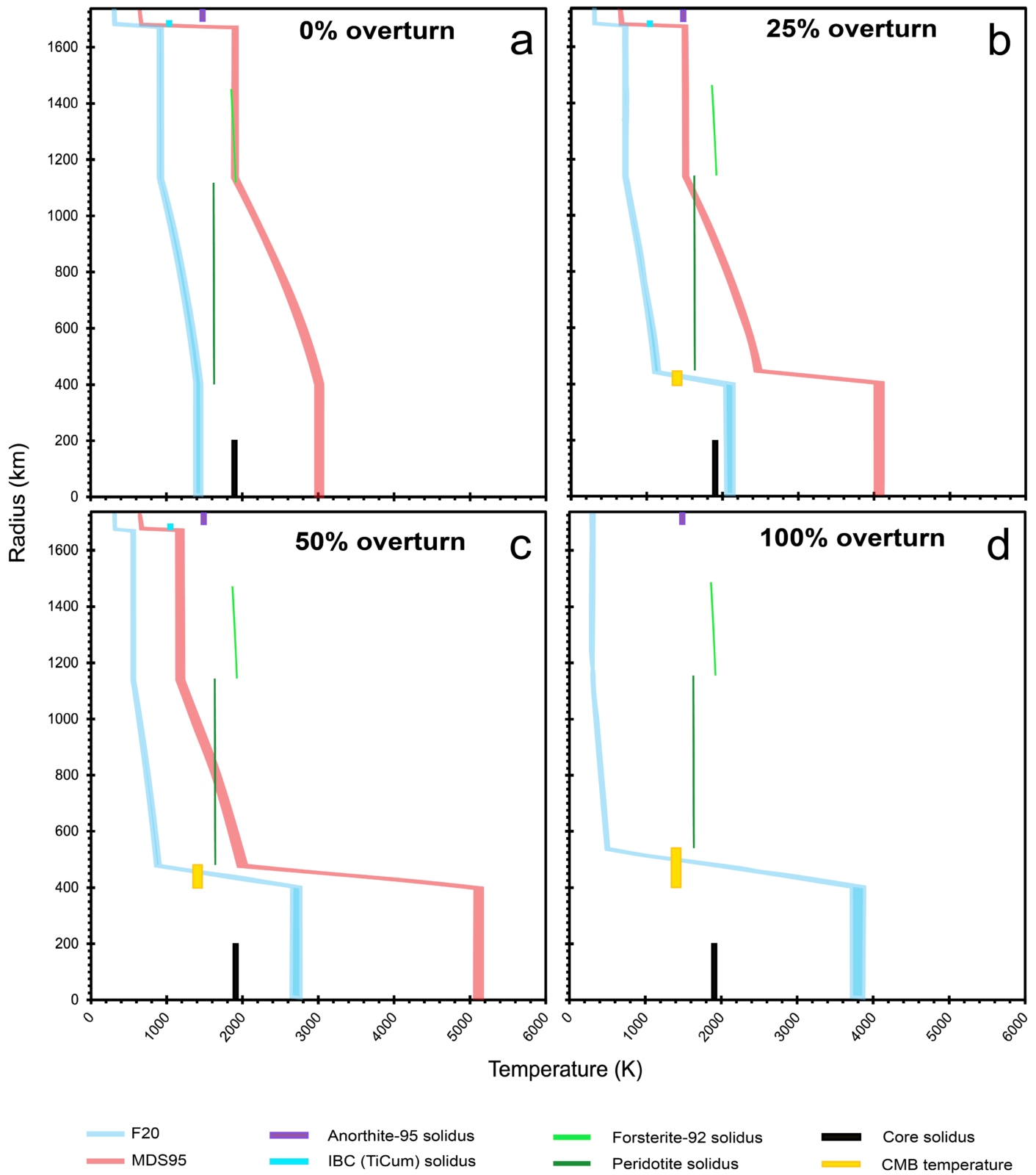
Figure 1: “Snapshot” selenotherms for the present-day lunar interior with varying overturn efficiencies (0 – 100%). Radioactive elements concentrations have been varied between those of McDonough and Sun, (1995) [red; MDS95 – estimates for Earth’s primitive mantle] and Faure et al., (2020) [blue, F20 – estimates for enstatite chondrites]. Melting temperature curves for lunar interior are shown for reference – Crust [anorthite-95; Yoder and Tilley, (1962)], upper mantle ilmenite bearing cumulates [TiCum; Van Orman and Grove, (2000)], dunite-bearing upper mantle [Forsterite-92; pMELTS], lower mantle [peridotite; Hirschmann, (2000)], and inner core solidus [γ-Fe; Konopkova et al., (2016)]. Temperature range required to sustain a small amount of magma at the core-mantle boundary [Mallik et al., (2019)] is marked in yellow.
We recreated the process of magma ocean cooling and crystallizing in our laboratory at the University of Arizona to study how these radioactive elements get distributed between minerals and liquid magma. Using the experimentally determined affiinity for the radioactive elements, we estimated how these elements are distributed accross the Moon’s internal layers. Thereafter, we calculated the heat they produce, and modeled how heat gets conducted within the lunar interior. Conductive heat transfer modeling maps out a “snapshot” of the interior of the Moon’s coldest possible temperature today. The selenotherm estimates are minimum because among different heat sources, only the thermal energy produced from the decay of these radioactive elements is considered. Feasibility of “snapshot” selenotherms were determined by a variety of mantle, crustal, and core melting temperatures. Most of the Moon’s interior is devoid of molten materials which helps justify the hotter selenotherms (see figure) which would cause vast portions of the interior to melt as unreasonable. The selenotherms from panel (a) and (b) in the figure best explain the current configuration of the lunar interior with a solid inner core and mantle while only a small amount of magma is present at depths of 1350 km from the surface.
The scientific study has been led by me (Arkadeep) and co-authored by my PhD advisor Ananya Mallik, collaborators Paul Bremner and Heidi Haviland from NASA’s Marshall Space Flight Center, Rick Hervig and Tyler Goepfert from Arizona State University, and Matthew Diamond from University of Illinois, Chicago. Our team made some key conclusions about the most reasonable selenotherms:
- The uranium and thorium in the Earth and Moon is dominantly sourced from a group of meteorites called enstatite chondrites.
- The process of mantle overturn was inefficient and only 0-25% of the late-stage cumulates sank to the core-mantle boundary.
Thus our research helps us piece together the building blocks that made up the Earth-Moon system and how the Moon’s interior evolved over billions of years. It also provides clues about similar processes on other planets and moons in our solar system.
References: McDonough, W. F., & Sun, S. S. (1995). The composition of the Earth. Chemical geology, 120(3-4), 223-253. Faure, F. (2020). Early silica crust formation in planetesimals by metastable silica-rich liquid immiscibility or cristobalite crystallisation: the possible origin of silica-rich chondrules. Scientific reports, 10(1), 4765. Yoder Jr, H. S., & Tilley, C. E. (1962). Origin of basalt magmas: an experimental study of natural and synthetic rock systems. Journal of Petrology, 3(3), 342-532. van Orman, J. A., & Grove, T. L. (2000). Origin of lunar high‐titanium ultramafic glasses: Constraints from phase relations and dissolution kinetics of clinopyroxene‐ilmenite cumulates. Meteoritics & Planetary Science, 35(4), 783-794. Hirschmann, M. M. (2000). Mantle solidus: Experimental constraints and the effects of peridotite composition. Geochemistry, Geophysics, Geosystems, 1(10). Konôpková, Z., McWilliams, R. S., Gómez-Pérez, N., & Goncharov, A. F. (2016). Direct measurement of thermal conductivity in solid iron at planetary core conditions. Nature, 534(7605), 99-101. Mallik, A., Ejaz, T., Shcheka, S., & Garapic, G. (2019). A petrologic study on the effect of mantle overturn: Implications for evolution of the lunar interior. Geochimica et Cosmochimica Acta, 250, 238-250.