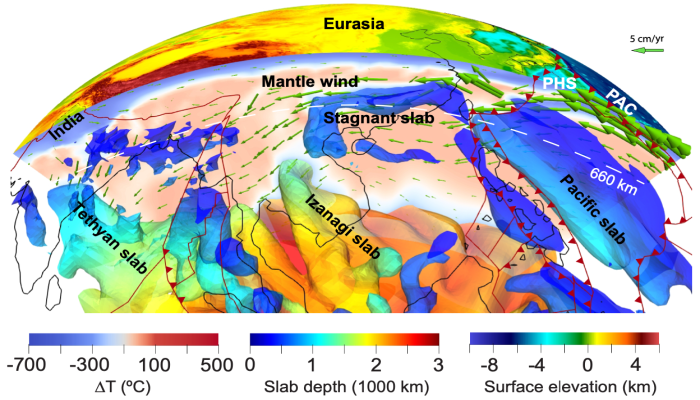
Mechanisms for stab stagnation are essential for understanding the mantle circulation patterns. This week, Diandian Peng, a graduate student from University of Illinois explores how dynamic pressure gradients can pull the slabs sideways stagnating them at the Mantle Transition Zone.
Seismic imaging provides critical constraints on the structure and evolution of subducted slabs. Numerous tomographic studies (Obayashi et al., 2013; Ritsema et al., 2011) showed that slabs could ultimately reach the lowermost mantle, thus supporting the notion of whole-mantle convection. However, it is unclear what controls the prominent horizontal displacement of slabs while descending. A particularly relevant question is why some slabs (e.g., Pacific slab beneath East Asia) seem to be stagnated within the mantle transition zone (MTZ) while other slabs are not? Traditional mechanisms for the stagnant slabs mainly focus on the vertical resistance force (Figure 1A). These include (1) the buoyancy anomaly associated with phase transformations of major mantle minerals, due to the negative Clapeyron slope of ringwoodite-bridgmanite transformation (Christensen & Yuen, 1985; Tackley et al., 1993) and/or delayed occurrence of such phase transformations (King et al., 2015); (2) radial viscosity variations with either a sudden viscosity increase into the lower mantle (Gurnis & Hager, 1988), or a thin low-viscosity layer right below the 660 km (Mao & Zhong, 2018); and (3) trench retreat, which is commonly attributed as an important reason for slab stagnation (Christensen, 1996). Recent studies with global mantle convection models discovered a new mechanism that highlights the lateral mantle flow instead (Figure 1B). The mantle flow induced by lateral gradients of dynamic pressure pulls the slabs away horizontally to form the stagnant configuration, as observed by Peng et al., 2021a and Peng et al., 2021b.
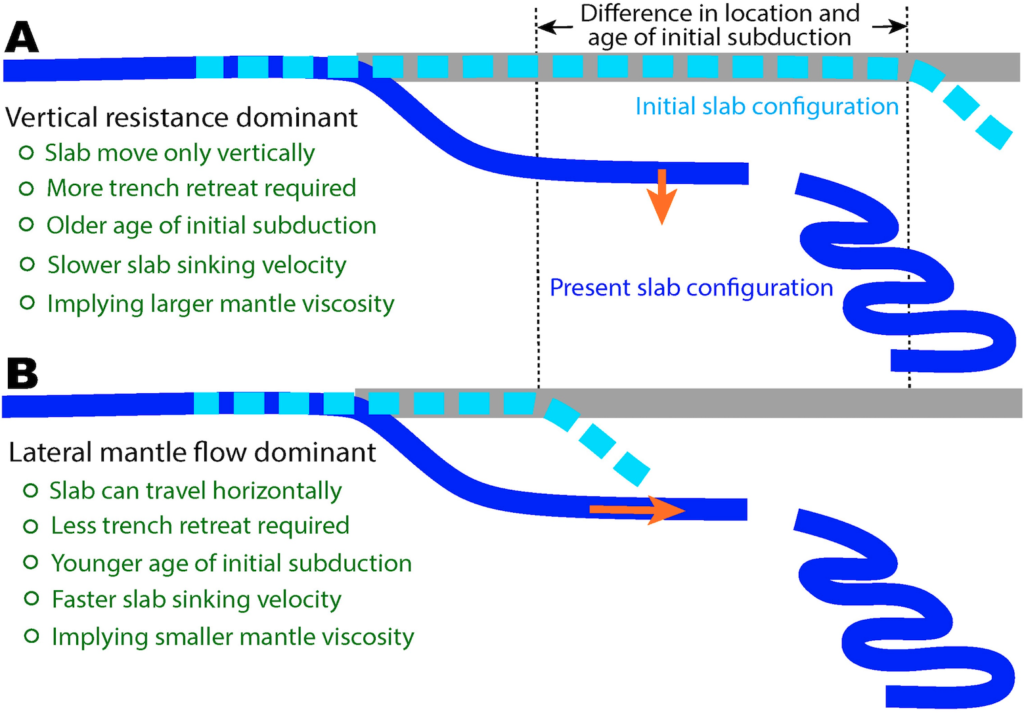
Figure 1. Two end-member views on how slabs sink through the mantle. (A) The traditional vertical-sinking slab hypothesis, where lateral slab displacement is solely due to trench retreat. (B) A new scenario where slabs can travel horizontally while sinking. This scenario requires much less trench retreat as that in (A). Multiple differences between A & B on fundamental mantle and tectonic implications are listed.
In a subduction system, the mantle below the subducting plate tends to experience a Couette-type flow driven by surface plate motion, characterized by decreasing horizontal velocity with increasing depth from the base of the plate. In contrast, the mantle below the overriding plate tends to display a trench-ward Poiseuille-type flow driven by the lateral pressure gradient originating from the mantle wedge (Hu et al., 2017). According to these global-scale convection models, continuous Mesozoic subduction progressively reduced the dynamic pressure within the mantle wedge below East Asia (Figure 2A-C) since a sinking slab depressurizes the mantle above. Prior to the arrival of the Izanagi-Pacific mid-ocean ridge (MOR) into the trench, the lateral pressure gradient excited a trench-ward Poiseuille-type flow beneath the overriding Asian plate. In contrast, beneath the subducting slab, the flow is Couette-type, suggesting that the surface plate motion drove the underlying mantle flow. In this case, the Izanagi slab behaved as a mechanical barrier that separated the flow field above it from that below. With time, the pressure gradient across the slab hinge grew strong enough to reduce the dip angle of the Izanagi slab, which eventually became flat at 70 Ma (Figure 2C). This newly discovered flat subduction explains the multiple East Asian tectonic events, including the unique lithospheric structure (Liu et al., 2021; Peng et al., 2021a).
After the Izanagi-Pacific MOR entered the trench around 55 Ma, the Izanagi slab started to detach from the surface plate. The gradual disappearance of the mechanical barrier (i.e., the strong slab) connected the low-pressure mantle above the Izanagi slab directly with the high-pressure asthenosphere beneath the sinking MOR. Consequently, the flow beneath the incoming Pacific Plate became dramatically different from that before. This is because the newly established sharp lateral pressure gradient across the MOR triggered a fast westward Poiseuille-type flow from the oceanic asthenosphere to the mantle wedge (Figure 2D). This westward flow originated from beneath both the subducting Pacific Plate and Philippine Sea Plate and was maintained throughout the Cenozoic (Figure 2D-E). This lateral flow acted as a regional mantle wind that carried the subducting Pacific and Philippine Sea slabs toward the west while the pressure gradient across the slabs lifted them and thus prevented them from entering the lower mantle. Carried by this mantle wind, the slab traveled a long-distance westward to form a >1500-km-long stagnant slab as observed beneath East Asia.
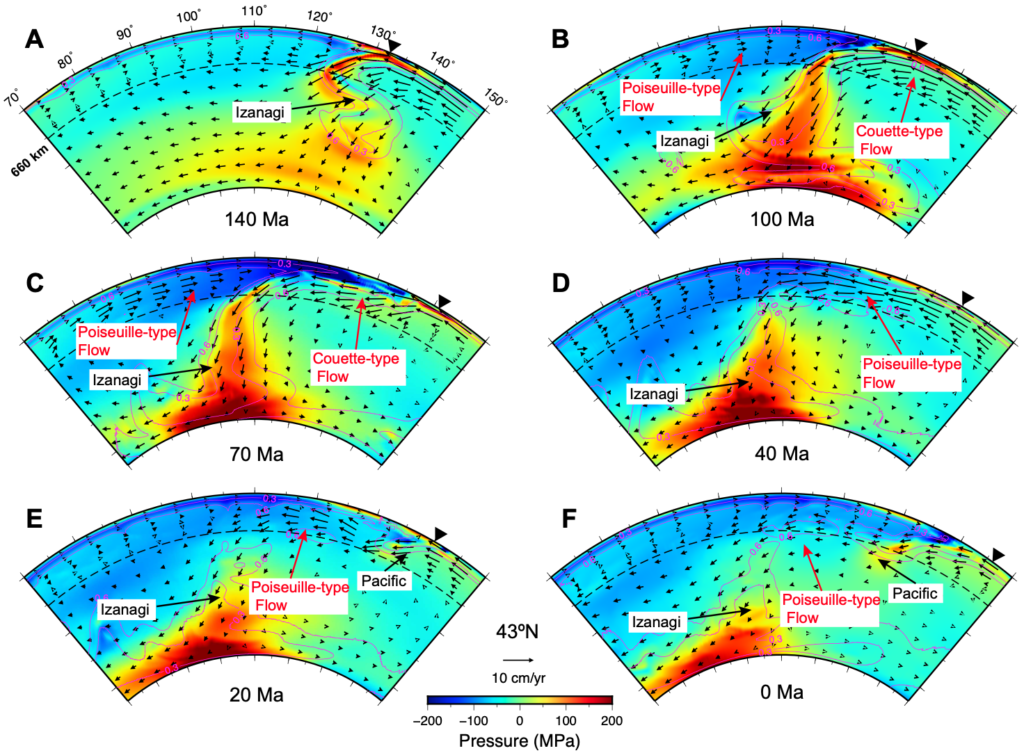
Modeled evolution of dynamic pressure and mantle flow (based on Peng et al., 2021b). (A-C) Prior to the subduction of the Izanagi-Pacific MOR, the low pressure above the Izanagi slab accumulated and led to the flat subduction of the Izanagi slab. (D-F) After the MOR subduction, the mantle flow pattern below East Asia changed from being eastward in the latest Cretaceous to westward in the Cenozoic. This mantle wind driven by the lateral dynamic pressure gradient promoted the formation of the stagnant slab.
Compared to the traditional mechanisms of slab stagnation which mostly focus on vertical resistances, the lateral mantle flow induced by dynamic pressure explains why the distribution of stagnant slabs is limited to certain regions like East Asia. While the vertical resistant forces are universal for all subduction zones, the formation of regional mantle winds requires a gradual build-up of dynamic pressure followed by a sudden pressure release during slab tearing or MOR subduction. The flat subduction of the Izanagi slab during the Mesozoic determined its landward shifted position inside the lower mantle during the Cenozoic, and the resulting pressure gradient tends to induce a westward mantle wind. This explains why only at limited locations seismic imaging detects stagnant slabs. The dynamic pressure might also help the MOR to subduct during the early Cenozoic. Young oceanic plates are more buoyant than the surrounding mantle and tend to resist subduction. After the MOR enters the trench, the formation of the Poiseuille-type flow below the western Pacific could actively entrain the buoyant MOR into the subduction zone during the early Cenozoic. Therefore, the landward mantle wind may also act as a generic driving force for ridge subduction.
References Christensen, U. R. (1996). The influence of trench migration on slab penetration into the lower mantle. Earth and Planetary Science Letters, 140(1–4), 27–39. https://doi.org/10.1016/0012-821X(96)00023-4 Christensen, U. R., and Yuen, D. A. (1985). Layered convection induced by phase transitions. Journal of Geophysical Research, 90(B12), 10291. Van der Hilst, R., & Seno, T. (1993). Effects of relative plate motion on the deep structure and penetration depth of slabs below the Izu-Bonin and Mariana island arcs. Earth and Planetary Science Letters, 120(3–4), 395–407. Hu, J., Faccenda, M., & Liu, L. (2017). Subduction-controlled mantle flow and seismic anisotropy in South America. Earth and Planetary Science Letters, 470, 13–24. King, S. D., Frost, D. J., & Rubie, D. C. (2015). Why cold slabs stagnate in the transition zone. Geology, 43(3), 231–234. Liu, L., Peng, D., Liu, L., Chen, L., Li, S., Wang, Y., et al. (2021). East Asian lithosphere evolution dictated by multistage Mesozoic flat-slab subduction. Earth-Science Reviews, 217(April), 103621. Obayashi, M., Yoshimitsu, J., Nolet, G., Fukao, Y., Shiobara, H., Sugioka, H., et al. (2013). Finite frequency whole mantle P wave tomography: Improvement of subducted slab images. Geophysical Research Letters, 40(21), 5652–5657. Peng, D., Liu, L., & Wang, Y. (2021a). A Newly Discovered Late‐Cretaceous East Asian Flat Slab Explains Its Unique Lithospheric Structure and Tectonics. Journal of Geophysical Research: Solid Earth, 126(10), 1–23. Peng, D., Liu, L., Hu, J., Li, S., & Liu, Y. (2021b). Formation of East Asian Stagnant Slabs Due To a Pressure‐Driven Cenozoic Mantle Wind Following Mesozoic Subduction. Geophysical Research Letters, 48(18), 1–10. Ritsema, J., Deuss, A., Van Heijst, H. J., & Woodhouse, J. H. (2011). S40RTS: A degree-40 shear-velocity model for the mantle from new Rayleigh wave dispersion, teleseismic traveltime and normal-mode splitting function measurements. Geophysical Journal International, 184(3), 1223–1236. Tackley, P. J., Stevenson, D. J., Glatzmaier, G. A., & Schubert, G. (1993). Effects of an endothermic phase transition at 670 km depth on spherical mantle convection. Nature, 361, 699–704. Zhong, S., & Gurnis, M. (1995). Mantle convection with plates and mobile, faulted plate margins. Science, 267(5199), 838–843.
Ufas Webmaster
Nice article. Thank you very much