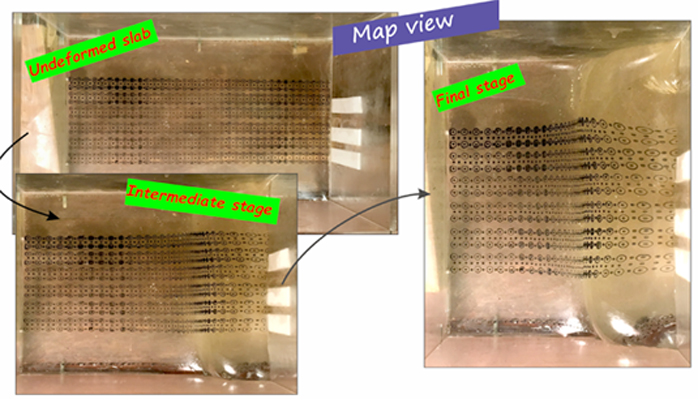
Accretionary prisms are wedge-shaped bodies of crustal rocks that are commonly known to have formed by accommodating the component of convergence along subduction margins. These prisms, primarily wedge shaped with lateral variations in topographic height from hinterland to foreland are commonly characterised by a gentle and uniform slope towards the frontal margin (for instance, the Himalayan fold-thrust belt has a uniform gentle slope of 4° towards the frontal margin). However, there are some accretionary wedges that are found to possess an unlike topographic curvature with an upward convexity (Fig. 1). The development of such an unusual topography remains to be discerned from convergent tectonic models. In this week’s News and views, Sreetama Roy, a PhD student from the University of Calcutta, will share her experimental works to understand the development of convex upward topography along the subduction zone.
In common know-how, the disposition of such convex-upward topographic curves may seem to be an unrealistic development resulting from exaggerated vertical build-up in a convergent deformational regime. However, none of the previous studies considered the role of decollement coupling in a subduction zone that might be crucial in developing such unusual topography. Basal decollements/detachments are a common feature of fold-thrust belts/accretionary prisms and are basal surfaces that generally dip at very low angles towards the interior of mountain belts and below which there is no deformation. My new crustal scale laboratory model observations coupled with the pre-existing theoretical analyses suggest that such an unconventional convex-upward topography is characteristic of strong basal decollement coupling beneath a belt of crustal rocks that comprise the accretionary wedge (refer to Roy et al., 2020 for detailed analyses and other structural insights on convergent tectonics).
Such crustal scale laboratory experiments commonly incorporate the viscous rheology of Polydimethylsiloxane or PDMS (Weijermars, 1986, a transparent silicone polymer used for simulating ductile regions within the crust). This material does not adhere to natural complexities, yet easily accommodates pervasive, distributed and spatially variable deformation, especially applicable to natural tectonic scenarios where deformation is dominantly controlled by fluid or thermally activated creep. With a viscosity and density in the order of 5×104 Pas and 965 kg/m3 respectively, PDMS models can suitably be scaled with crustal magnitudes for a qualitative understanding of the balance between lateral compressive stress and gravity forces within the deforming wedge model. To generate compressive stress, a moving piston at a constant speed (~1.97 × 10-4 ms-1) was used and the convergence rate was kept uniform to annul the effects of rate dependence on viscous deformation. From these models, we can understand how the geometries along accretionary prisms develop and also gain insights on the role of basal decollement coupling in convergent deformation mechanics.
I assumed a length ratio, (nature/model) in the order 106 and density ratio, ~ 2.8 (calculated) for appropriate scaling of models to natural prototype. For realistic approximation, only the ratio between viscous and gravitational forces was considered, given that inertial forces are negligible in my experiments.
These experiments were performed in a natural gravity field (Fig. 2) and thus the force balance equation may be expressed as follows-
ηRέR=ρRlR ...(eq 1)
where ηR & έR are the viscosity and strain rate ratios between nature and model respectively. As the strain rate ratio (έR) is an inverse of the time ratio (TR), eq.1 given above can be simplified as follows:
ηR = ρRlRTR ... eq (2)
From eq.2, the viscosity ratio (ηR) was quantified to be in the order of ~ 5.6 × 1017. This implies that the chosen model material with a viscosity (ηm) in the order of 104 Pas represents natural crustal rocks having a viscosity (ηn) in the order of ~ 5.6 × 1021 Pas and is adequately scaled to replicate natural crustal rocks that comprise the accretionary wedges.
Strength of coupling of the basal decollement is a crucial mechanical parameter that controls the propagation of deformation and its mechanics in a progressively deforming fold-thrust belt/accretionary prism. My models including a uniformly strong decollement have shown that the wedge shaped crustal structure is characterised by a steep surface slope over a relatively narrow wedge (Fig. 3). In the mature stages of wedge evolution (for more clarity on stages of evolution in viscous wedge tectonics, refer to Roy et al., 2020), the steepness of the wedge profile is relatively slackened due to the lateral flow of wedge material from the hinterland towards the foreland due to significant difference in elevation between the two regions over a narrow deformed width. The shape of accretionary wedges deduced theoretically from the steady-state circulation of “fluid” sediments is convex upwards (Emerman and Turcotte, 1983). The best-fit approximations of topographic wedge profile are in cognitive agreement with the bathymetric profiles across the Kuril and Ryukyu accretionary wedges (Fig. 4) that have been acquired from cruise 1219 of R/V “Conrad” and cruise 1217 of R/V “Conrad” respectively.
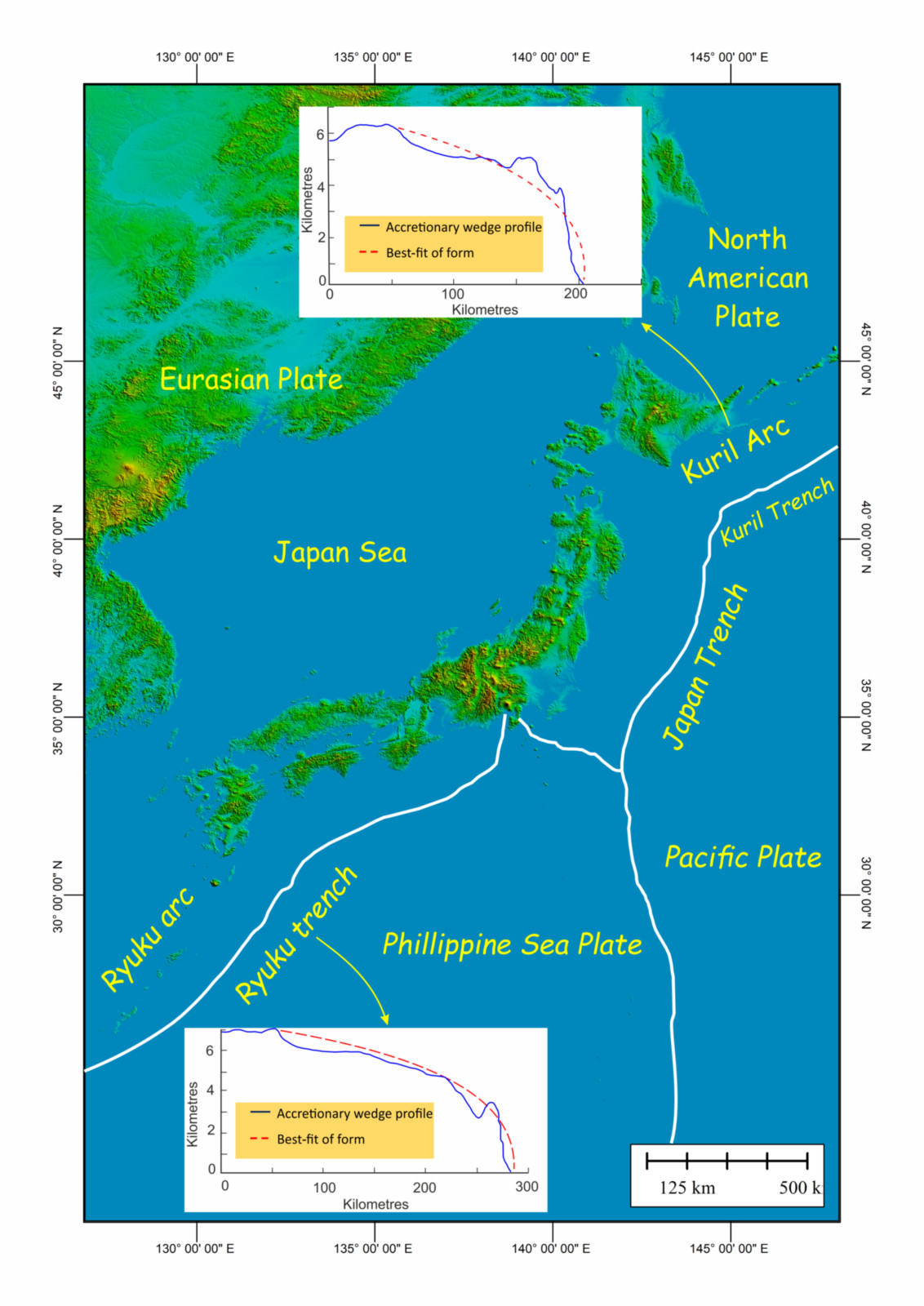
Fig. 4 Tectonic map in and around Japan Sea (modified after Iwasaki et al., 2019) and graphs in inset modified after (Emerman and Turcotte, 1983).
My model results corroborate these observations by replicating the typical wedge profile. In vertical cross-sections they reveal significant first-order insights on the mechanics of morpho-metric evolution of such large-scale upward convexity (Fig. 3). These results also confirm the pivotal role of a strongly coupled decollement in steering the deformation to occur in such a complex manner in the hinterland zone at experimental strain rates scaled to standard natural rates in the order of 10-15 s-1. In a nutshell, a simple fluid model simulating crustal rocks when deformed under uniaxial compression over a strongly coupled decollement, leads to the development of wedge shapes that contend with the accretionary profiles across several wedges in natural subduction zones.
Alternatively, frontal propagation of deformation within the wedge occurs at a significantly slow rate, thereby accumulating an unrealistically high magnitude of strain at the topographic slope break. This zone can be demarcated as a potential site for large scale thrusting event in orogenic belts (Fig. 3). The simultaneous rotation of strain ellipses on the XZ section and decrease in wedge slope is entirely attributed to the activation of gravity flow in the hinterland. Therefore the episodic events of gravitational collapse and subsequent spreading of overlying crustal rocks within the Nepal Himalaya are most likely to have occurred when the Main Himalayan Thrust/MHT (basal decollement) underlying the Nepal Himalaya is strongly coupled.
References: Cardozo, N., Allmendinger, R. W. (2009). SSPX: A program to compute strain from displacement/velocity data. Computers Geosciences, 35(6), 1343-1357. Emerman, S. H., Turcotte, D. L. (1983). A fluid model for the shape of accretionary wedges. Earth and Planetary Science Letters, 63(3), 379-384. Iwasaki, T., Tsumura, N., Ito, T., Arita, K., Makoto, M., Sato, H., ... & Suzuki, K. (2019). Structural heterogeneity in and around the fold-and-thrust belt of the Hidaka Collision zone, Hokkaido, Japan and its relationship to the aftershock activity of the 2018 Hokkaido Eastern Iburi Earthquake. Earth, Planets and Space, 71(1), 1-19. Roy, S., Bose, S., Saha, P. (2020). Spatial variations of ductile strain in fold-and-thrust belts: From model to nature. Journal of Structural Geology, 134, 104012. Weijermars, R. (1986). Flow behaviour and physical chemistry of bouncing putties and related polymers in view of tectonic laboratory applications. Tectonophysics, 124(3-4), 325-358.