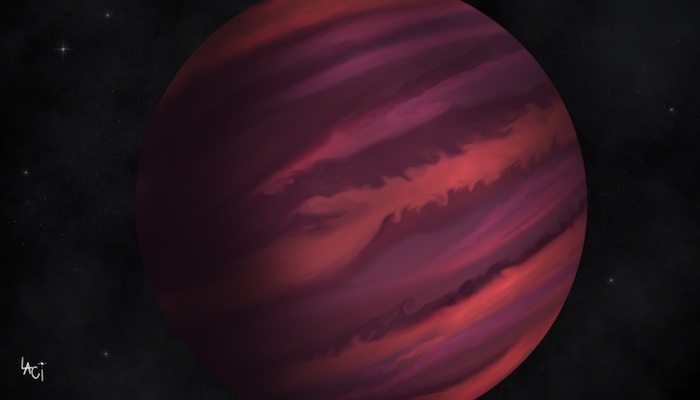
The universe is made up of stars and planets but have you ever wondered if there is anything in between? This week, Laci Brock, a PhD student from the University of Arizona’s Lunar and Planetary Laboratory, takes us on a journey into this murky region of stellar classification…
Four years before the cult classic science fiction show Star Trek debuted on television in 1966, astrophysicist Dr. Shiv Kumar was on a mission to explore strange new worlds of his own, predicting objects unlike anything we had yet discovered. Dr. Kumar’s theory described an entirely new class of stellar bodies in the Universe that should exist according to the laws of physics (Joregens, 2016). The idea was simple enough: if the mass of a star is below the minimum mass required for hydrogen fusion, this would result in a gaseous object not quite massive enough to become a main sequence star but perhaps too massive to be considered a planet. Enter brown dwarfs. The existence of these astronomical objects was not observationally confirmed until over 30 years after the idea was first proposed.
Stars are traditionally classified into groups depending upon their mass, temperature, and spectral features. Before brown dwarfs infiltrated the scene, seven letters of the alphabet were used to categorise stars created by astronomer Annie Jump Cannon: O, B, A, F, G, K, M (Figure 2). O-type stars are the most massive and hottest stars with effective temperatures exceeding 30,000 K. They radiate most of their energy in the ultraviolet portion of the spectrum making them appear blue in colour. M-type stars, often referred to as red dwarfs, are the least massive stars with cooler temperatures near 3000 K. Our Sun falls roughly in the middle as a G-type star.
Brown dwarfs remained elusive for so long because they are more difficult to detect than stars. Oh and also, they’re not brown. Brown dwarfs emit most of their energy in the infrared portion of the electromagnetic spectrum – the warmest would likely glow an orangish-red and the coolest more of a magenta hue (Figure 1). A combination of the world’s largest ground-based telescopes and space telescopes are required to detect even the brightest brown dwarfs. Thousands have been detected thus far, and it is thought there are likely billions of these low-mass objects lurking in the Milky Way, perhaps as many as there are stars.
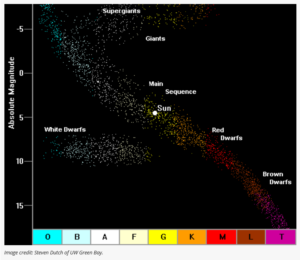
Figure 2: O-type stars are the most massive and the Sun is a G-type star. Brown dwarfs plot along the Hertzsprung–Russell diagram on the far right. Note that Y dwarfs are not included in this diagram.
Brown dwarfs are composed of mostly hydrogen and helium and form like stars via the gravitational collapse of molecular clouds. Stars generate thermal energy in their cores from nuclear fusion, sustaining a majority of their lifetime on the main sequence in hydrostatic equilibrium – a perfect balance between inward self-gravity and outward thermal pressure. Brown dwarfs range from about 13 to 80 times the mass of Jupiter but never reach the masses, and thus internal temperatures, required to fuse hydrogen into helium like stars. As a result, brown dwarfs are unable to stop gravitational contraction and develop cores supported by electron degeneracy pressure. Eventually, brown dwarfs fizzle out and become ‘failed stars’, leaving only a faint glow leftover from formation as they cool, contract, and age.
But what’s so special about these stellar rejects? Brown dwarfs are essentially the missing link between high-mass planets and low-mass stars. They encompass effective temperatures from warmer, star-like temperatures of ≤ 3000 K to those as cool as ~ 600 K, roughly the perfect temperature to cook a brick oven pizza. The discovery of objects across a diverse range of temperatures and masses required an extension to the existing stellar classification system (remember OBAFGKM?). Three new spectral types – L, T, and Y – were added to accommodate brown dwarfs cooler than the latest M-type red dwarf stars (Kirkpatrick, 2005).
Are you familiar with the sulphuric acid clouds of Venus or perhaps the enticing ammonia clouds of Jupiter? Brown dwarfs have unique clouds of their own composed of minerals similar to those found in the Earth’s mantle. Atmospheres of L-type brown dwarfs are dominated by thick, dusty condensate clouds composed of silicates and materials such as iron (Fe), corundum (Al2O3), enstatite (MgSiO3), and forsterite (Mg2SiO4). Thick condensate clouds begin to disappear below the photosphere (i.e., the observable `surface’ of a star) in the atmospheres of cooler T dwarfs. Although the coolest brown dwarfs are sometimes referred to as ‘cloud-free’, they probably have optically thin or inhomogeneous clouds of condensates such as Na2S, KCl, ZnS, MnS, and Cr (Morley et al., 2012).
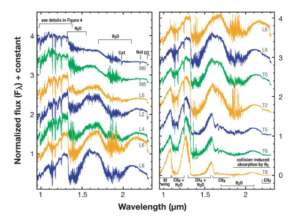
Figure 3: An example of the near-infrared spectral sequence of mid-M through late-T spectral types. Figure from Kirkpatrick (2005).
Continued photometric and spectroscopic studies will help us to better understand the lowest-mass products of star formation. Brown dwarf evolution is complex. Molecules in the atmospheres of brown dwarfs have opacities that can vary strongly with wavelength (Marley and Robinson, 2015), making it challenging to fit spectral energy distributions with global stellar atmosphere models. Learning how intertwined changes in luminosity, atmospheric cloud opacity, and age impacts the spectral energy distribution is tricky to unravel. One approach to this problem is to study brown dwarf binaries with empirically determined properties, such as mass, and use this information to test model predictions. In my recent paper, we explore how changes in cloud pressure and particle size in atmosphere models contribute to observed luminosity changes in a sample of brown dwarf binaries with known mass (Brock et al., 2021). We find that L dwarfs are dominated by higher altitude clouds with submicron particles, while T dwarfs have clouds deeper in the atmosphere with particle sizes ≥ 1 micron.
Brown dwarfs are certainly intriguing and showcase the diversity of objects in the Universe. The addition of new, powerful telescopes, such as The James Webb Space Telescope, will push our ability to detect even more brown dwarfs in the solar neighbourhood.
References
Brock et al. (2021). Cloud Properties of Brown Dwarf Binaries Across the L/T Transition. The Astrophysical Journal, 914(2), 124. doi: 10.3847/1538-4357/abfc46
Joregens, V. (2016). 50 Years of Brown Dwarfs. Springer International Publishing.
Kirkpatrick, J. D. (2005). New Spectral Types L and T. Annual Review of Astronomy and Astrophysics, 43(1), 195-245. doi: 10.1146/annurev.astro.42.053102.134017
Marley, M. S. and T. D. Robinson. (2015). On the Cool Side: Modeling the Atmospheres of Brown Dwarfs and Giant Planets. Annual Review of Astronomy and Astrophysics, 53(1), 279-323. doi: 10.1146/annurev-astro-082214-122522
Morley et al. (2012). Neglected Clouds in T and Y Dwarf Atmospheres. The Astrophysical Journal, 756(2), 172. doi: 10.1088/0004-637X/756/2/172