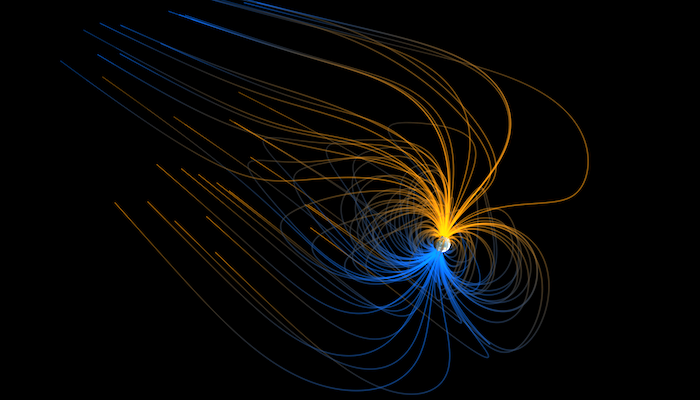
Geodynamics does not stop at the core-mantle boundary – the Earth’s outer core is a truly dynamic geosystem. This week Stefano Maffei (Research fellow) and Chris Davies (Associate Professor) from the School of Earth and Environment at the University of Leeds tell us more about the possible interplay between mantle and core and some of the remaining mysteries of the Earth’s magnetic field generation.
Studying the Earth’s geomagnetic field is truly fascinating – and highly multidisciplinary. Behind a single magnetic observation at the Earth’s surface lie signals from all regions of the planet and beyond: (1) the outer core, where the geomagnetic field is generated; (2) mantle and crust, where inhomogeneities in electrical conductivity and magnetised rocks alter the magnetic signal; (3) the atmosphere, magnetosphere and ionosphere, where trapped solar particles generate observable electrical currents; and (4) the Sun, whose extreme activity can cause geomagnetic storms with the potential of shutting down national power grids. This complexity cannot be tackled all at once, it is necessary to isolate phenomena and dissect them independently from everything else. This is challenging enough!
Take the Earth’s outer core: the main component of the geomagnetic field is generated here through the geodynamo process in which fluid motions twist, squeeze, and amplify an initial magnetic field (Figure 1) – under the right conditions, of course. To reproduce the geodynamo, the most common approach is to focus on magnetic field generation in a homogeneous, incompressible fluid set in motion by a hot bottom boundary (representative of the inner-outer core discontinuity). Reproducing an Earth-like geodynamo in numerical simulations is extremely challenging, even under these assumptions, which are not very realistic. Both the core’s inner and outer boundary are likely heterogeneous, so we neglect something potentially important to understand magnetic field generation on Earth.
It has long been observed that both the inner core and the lower mantle have a thermochemical structure that most likely results in inhomogeneous driving of convection in the core and the generation mechanism of the geomagnetic field [e.g., Aubert et al., 2013; Mound et al., 2019]. Seismic wave propagation through the Earth’s interior revealed two Large Low Velocity Provinces (LLVP, Figure 2) sitting at antipodal locations on the core-mantle boundary below Africa and the Pacific [e.g., Lekic et al., 2012]. These structures – sometimes called Jason & Tuzo (better than LLVP, isn’t it? – are conventionally assumed as anomalously hot and may thus extract heat from the core differently than the rest of the mantle. This potentially alters the dynamo process below. If also compositionally anomalous, Jason & Tuzo may furthermore influence outer core dynamics, for example through chemical or electromagnetic interactions. They probably also alter core-mantle boundary topography.
The big question is: are such effects detectable in geomagnetic observations?
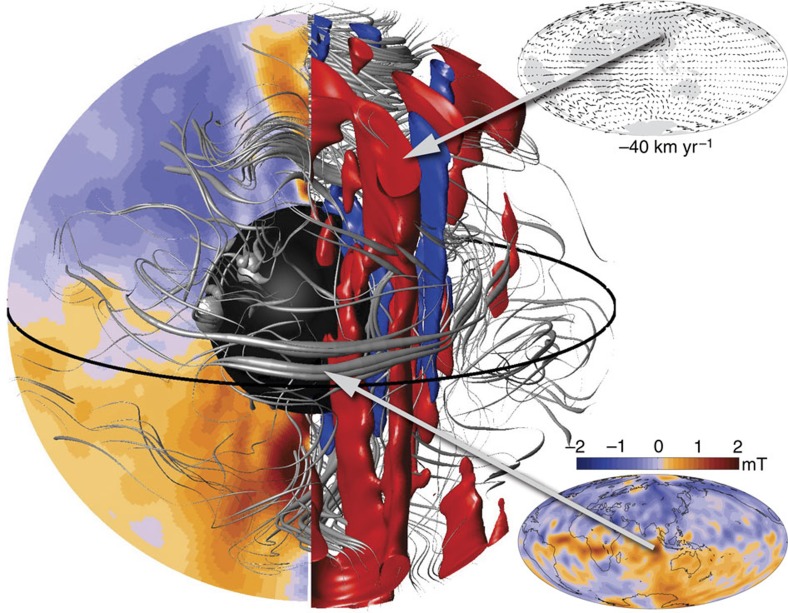
Figure 1: The estimated magnetic field and flow within the Earth’s core in 2015 from a geodynamo model [Aubert et al., 2013]. Orange and blue contours show the averaged intensity of the radial magnetic field within the shell, and at the core surface in the inset. The red and dark blue surfaces denote constant axial flow velocity to demonstrate columnar convection. Field lines within the shell have thickness proportional to magnetic energy. The inner core is black and the core–mantle boundary is transparent. From Finlay et al. (2016).
Let us focus on one example, the motion of the geomagnetic poles during geomagnetic reversals. It seems reasonable to look for detectable effects of lower mantle heterogeneity in the most drastic geomagnetic variations that are known to have happened. Unfortunately (well, actually ‘luckily’, and let’s keep it that way!), we have never directly observed a reversal, so we have to rely on indirect observations. To measure the magnetic field during past reversals we need to find the right type of depositional sequences that formed at the right times, involving the right kind of magnetic minerals. Presently available records are unevenly distributed across the globe. From these records, we can estimate the path that geomagnetic poles take during a reversal by calculating something called the Virtual Geomagnetic Pole (VGP): the location of the North Pole of a giant magnet bar placed at the centre of the planet that reproduces the magnetic measurements at the given location. It turns out that the VGP paths calculated from different locations mostly collapse along two preferential routes, approximately along the Eastern and Western boundaries of the Pacific Ocean [Laj, 2011]. Given that the geomagnetic field is not exactly the same as that produced by a magnet bar – in particular not during a reversal – one would expect the VGPs measured from different locations to follow no preferred path, especially not over multiple reversals event. But they do, and this indicates that something imposes a preferential orientation to the fluid core, which we do not expect from a well-mixed fluid confined between two uniform thermostats. Enter: Jason and Tuzo!
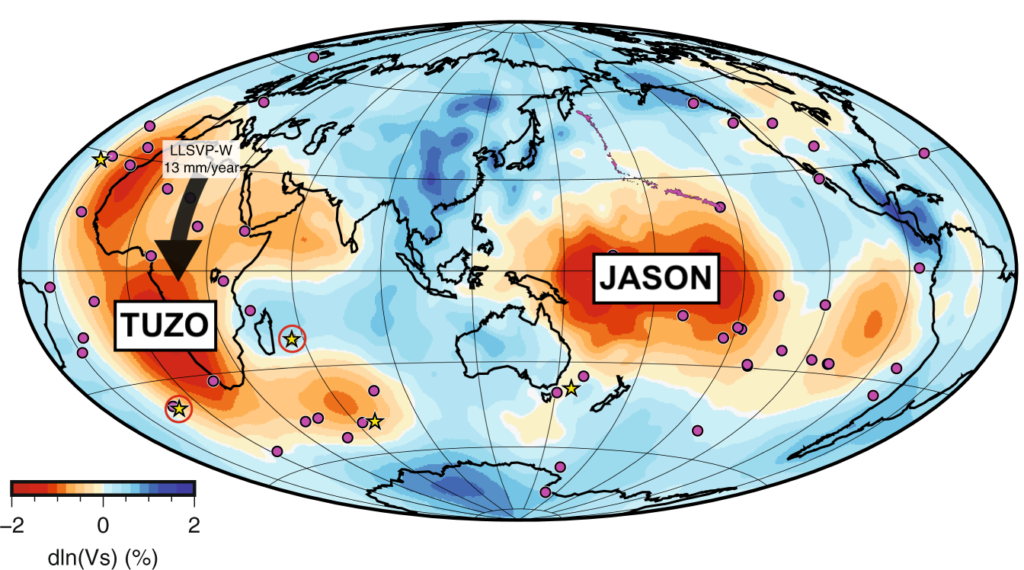
Figure 2: The two large low shear velocity provinces (Tuzo & Jason) as inferred from seismic tomography. Shown is the SEISGLOB2 tomographic model of shear wave velocity [Durand et al., 2016] at 2700 km depth. Circles and stars show different classes of hotspots. From Bono et al. (2019), see there for further details.
Perhaps – or probably? – the explanation is a combination of all these effects. The jury is still out, perhaps for a long time, given the difficulties in simulating the geodynamo and in quantifying key properties of the lower mantle. Also, here is a call for caution that some studies suggest: if we sampled the paleomagnetic field in a different way, perhaps from more evenly distributed locations around the globe, we may see a weaker preference for VGP paths during reversals. Kind of a let-down, isn’t it? What is sure is that more work is needed – using observational, theoretical and numerical methods – to confirm the preferred VGP paths and to understand what they mean for the Earth. It’s a long way to go, and with so much new to explore. One key thing we have learned already is that mantle and core do not just do their own things, but interact with each other.
How exactly remains a well-hidden secret of our planet Earth – yet!
Further Reading
Aubert, J., Finlay, C. C., & Fournier, A. (2013). Bottom-up control of geomagnetic secular variation by the Earth’s inner core. Nature, 502, 219-223.
Bono, R.K. Tarduno, J.A. & Bunge, H.-P. (2019), Hotspot motion caused the Hawaiian-Emperor Bend and LLSVPs are not fixed, Nature Communications, 10, 3370.
Calkins, M. A., Noir, J., Eldredge, J. D., & Aurnou, J. M. (2012). The effects of boundary topography on convection in Earth′s core. Geophysical Journal International, 189, 799-814.
Costin, S. O., & Buffett, B. A. (2004). Preferred reversal paths caused by a heterogeneous conducting layer at the base of the mantle. Journal of Geophysical Research: Solid Earth, 109, B06101.
Durand, S., Debayle, E., Ricard, Y. & Lambotte, S., Seismic evidence for a change in the large-scale tomographic pattern across the D” layer. Geophysical Research Letters, 43, 7928–7936.
Finlay, C.C., Aubert, J. & Gillet, N. (2016), Gyre-driven decay of the Earth’s magnetic dipole, Nature Communications, 7, 10422
Kutzner, C., & Christensen, U. R. (2004). Simulated geomagnetic reversals and preferred virtual geomagnetic pole paths. Geophysical Journal International, 157(3), 1105-1118.
Laj, C. (2011). GEOMAGNETIC FIELD, POLARITY REVERSALS. Encyclopedia of Solid Earth Geophysics, 386.
Lekic, V., Cottaar, S., Dziewonski, A., & Romanowicz, B. (2012). Cluster analysis of global lower mantle tomography: A new class of structure and implications for chemical heterogeneity. Earth and Planetary Science Letters, 357, 68-77.
Mound, J., Davies, C., Rost, S., & Aurnou, J. (2019). Regional stratification at the top of Earth’s core due to core–mantle boundary heat flux variations. Nature Geoscience, 12(7), 575-580.
monkeytree
Do you think it possible that the magnetosphere migration speeds up cyclically on a seven hundred year period? Could the wave manipulation come from within (core dynamics), or from outside by interactions with other energy fields?
There is a thought that major civilizations rise and fall on seven hundred year cycles. The evidence of these civilizations prove the times of abundance when cultural expression would have developed. These would have been times of plenty between the warfare. These times of plenty would depend on extra heat.
Could this periodic heating be a type of induction heating formed by extra wave friction that results in pulses of heat expulsion on a seven hundred year cycle?
Has anyone compared the magnetic signature of these artifacts of different civilizations? Possibly many of the objects were dated by magid. This periodic cycling would have slowed core cooling and enabled the present ecology, as well the formation of life on earth.