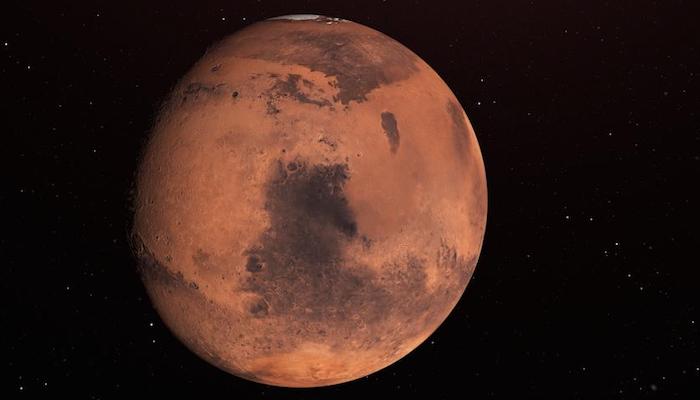
This week Ana-Catalina Plesa, Junior Research Group Leader at the German Aerospace Centre (DLR) in Berlin/Germany takes us on a journey to Mars. Enjoy the read about how spacecraft observations and numerical models provide InSight into the deep enigmas of the “red planet”.
Over the course of its evolution, Mars has accumulated heat in its interior arising from the planet’s formation, differentiation, and the decay of radioactive isotopes. The heat is eventually transported to the surface and lost to space as the planet cools. The planetary interior acts as a huge heat engine that converts heat into work, which is expressed in the form of volcanoes and tectonics at the surface. Mars’ formerly active, but now deceased core dynamo that once generated a magnetic field is another feature of that.
Numerical geodynamical models play a key role in understanding these processes controlling the interior evolution of rocky planets, but constraints are needed. Until recently, those were rather indirect for the interior of Mars, as a planet’s interior is most difficult to observe. But then the InSight mission (Interior Exploration using Seismic Investigations, Geodesy and Heat Transport) successfully landed on Mars – at “Homestead Hollow” in the Elysium Planitia region. Onboard is the most comprehensive set of geophysical instruments ever deployed on Mars with which InSight is investigating Mars’ interior engine since November 2018.
InSight is equipped with a seismometer (SEIS) to listen to the noises of the planetary engine, a heat flow probe (HP3) to measure how much heat is lost to space, a Doppler effect experiment (RISE) to track how Mars wobbles in its rotation, and additional instruments for atmospheric science and magnetic field investigations. The mission aims at placing constraints on the structure and thermal state of Mars’ interior, to understand its evolution and how all rocky planets including the Earth have formed.
Unlike the Earth, Mars does not possess plate tectonics, still its surface reveals some of the most spectacular volcanic and tectonic features in the Solar System. Large volcanic provinces such as Tharsis and Elysium – active over most of Mars’ history – show lava flows as young as a few million years and bear witness to the planet’s interior activity. Geodynamical models (Figure 2) of Mars’ thermal evolution suggest that interior magmatic activity may still be ongoing. In particular at Tharsis, a thick insulating crust keeps the interior warm and provides favorable conditions for mantle melting until the present day.
Pre-mission studies, partly based on geodynamical modeling, suggested that Mars’ level of seismicity should exceed the Moon’s, but is lower than Earth’s. Mars’ current geological activitiy has been unequivocally confirmed by InSight’s seismometer SEIS. Nearly 400 seismic events are listed in the latest Marsquake catalog. Two of these occurred in the Cerberus Fossae region, an extensive fracture system, thought to have been created by volcano-tectonic processes. The region shows lava flows as young as 10 Ma – rather young, given that most of the Martian surface is older than 3 billion years.
A first evaluation of the seismic activity of Mars revealed that the planet shakes more often than expected – but less severe. The largest Marsquake recorded by InSight has a magnitude around 4, not quite enough to probe the planet’s deep mantle and core, but sufficient to start placing constraints on the thickness of the Martian crust. The latter will provide unprecedented constraints on the level of volcanic activity that the planet has experienced. This is directly linked to mantle differentiation and magmatic degassing processes that occurred during the thermochemical history.
The heat flow probe (the mole) encountered problems shortly after it started burying itself by hammering into the ground in early 2019. Soon it became clear that the mole did not have enough friction on its hull to balance the recoil from hammering and advance underground. At Homestead Hollow, the regolith sand is apparently covered by an unusually thick (some 10s of cm) duricrust of chemically cemented sand. Due to its internal cohesion, that duricrust provides less friction than cohesionless (dry) sand for which the mole was designed. If the mole can even punch a hole in the crust as it did, the friction will vanish altogether for part of its hull length. At the time of this writing, the mole aided by the robotic arm is about 1 centimeter below the surface and it is hoped that it may soon progress on its own. See here for updates on the mole’s journey down to the desired depth of 3+ meters.
In the absence of direct heat flow measurements, constraints on the thermal state of Mars’ interior can be placed via the elastic thickness of the lithosphere – the part of the planet’s outer shell that deforms elastically when subjected to loads such as volcanoes or polar caps. Think of a jelly that deforms under the weight of a cookie on top! At the North pole of Mars, elastic thickness has been estimated from the surface deflection below the ice cap. The limited deflection measured by a radar penetrating the ice indicates a thick, cold lithosphere there. This places strong constraints on the thermal evolution of Mars and requires more than 50% of the radioactively generated heat to be produced within the Martian crust!
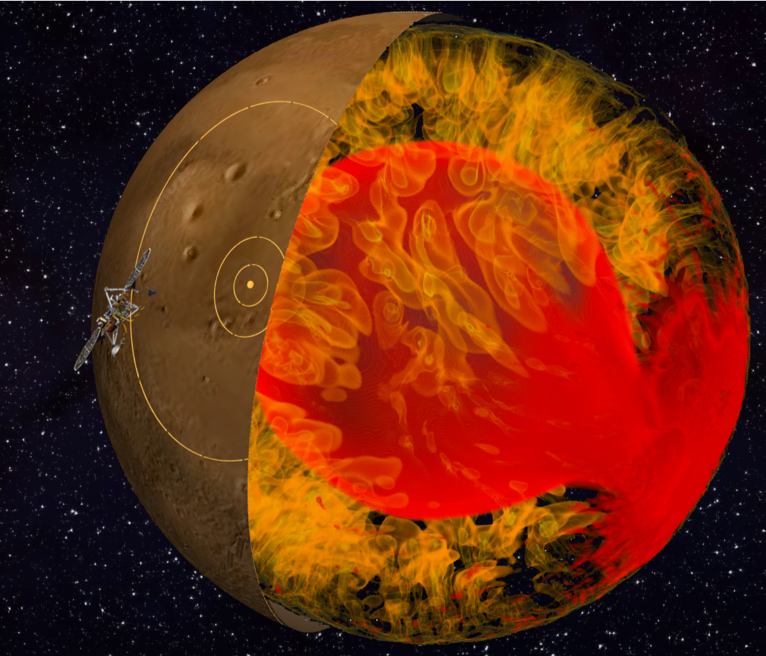
Figure 2: Snapshot from a geodynamic evolution model of Mars. The circles indicate seismic waves dispersing from Cerberus Fossae, a young fracture system associated with the origin of two seismic events recorded by InSight. Credit: A.-C. Plesa/DLR/NASA/JPL-Caltech.
Geodynamical models suggest that Mars’ core may be entirely liquid today. These model results will be put to test by the RISE experiment. RISE uses the X-band radio antenna located on the lander’s deck to accurately measure the nutation of the planet (wobbles of the rotation axis) by analyzing Doppler shifts in the radio signals used to communicate with the lander. This will help to determine the current state of the core (liquid or partially solid?): an entirely liquid core would cause Mars to wobble more than a solid one – much like a raw egg wobbles more than a hard boiled one when spun. This opens new avenues to understand core evolution, the early episode of magnetic field generation in Mars’ history, whose fingerprints are recorded by crustal rocks, and why the dynamo has stopped operating roughly 500 million years after planet formation.
And there is more to come: A larger quake capable of probing Mars’ interior down to the core, much like an X-ray travelling through different layers of tissue, may be just around the corner. The efforts of bringing the mole below the surface may soon be deservedly rewarded with the mole hammering on its own and providing the first direct heat flow measurement on Mars. So far, we have just scratched the surface of Mars, but now we are beginning to look deeper into its interior to understand the processes that have shaped the history of our planetary neighbor.
Imagine how much more there is to discover!