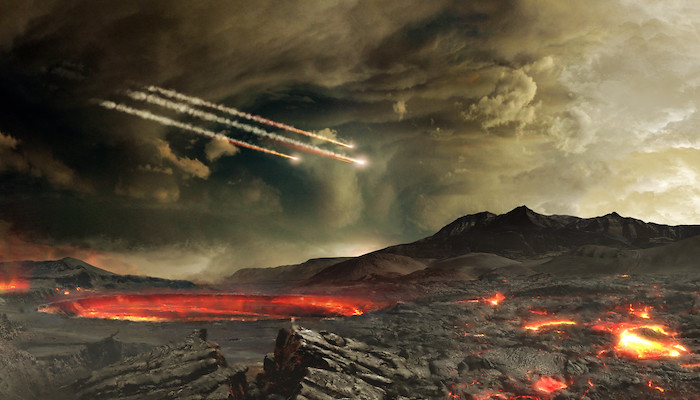
Today we know the Earth as a tectonically busy planet, shaped by mantle convection and plate tectonics. But this is nothing compared to the earlier phases of planetary evolution. This week, Fabio Capitanio (ARC Future Fellow and Associate Professor at the School of Earth, Atmosphere and Environment at Monash University, Australia) takes us on a modelling tour back to a time when the Earth’s tectonics functioned differently and quite hectically, yet produced something stable that is preserved until present.
The formation of the atmosphere, ocean deeps and mountain highs on our planet are understood to be the result of the peculiar evolution of the Earth’s tectonics. Throughout 4.5 billion years this went through different modes – or regimes – that operated differently from modern plate tectonics. The secrets of this evolution are kept in the continents, especially in the cores of cratons, the most stable and durable pieces of lithosphere that ever appeared. However, rocks created in the early Earth times are only sparsely preserved and – thus far – a conclusive understanding of our planet’s infancy has been elusive.
Continental cratons provide evidence of very active tectonics in the Hadean and Archean (> 3 billion years ago) that lead to the formation of land and to the evolution and diversification of species. Understanding the mechanisms of continent emergence therefore has the potential to answer some unresolved questions about this period, which displays enigmatic contrasting characteristics. Observed features and inferred processes are either reconducted to a modern style of plate tectonics or, instead, are explained by a rigid lithosphere with negligible horizontal motions and no real margins – quite the opposite of plate tectonics at present, right?
The early Earth’s tectonic mode has puzzled the geodynamics community for quite a while, yet no firm concensus has been found. While vigorous convection in a hotter Earth is an acknowledged result of larger internal radiogenic heat production in the mantle, how thick and rigid plates formed above such young, hot mantle and then disappeared leaving way to the transition to plate tectonics remain outstanding questions (Korenaga, 2006; Lenardic, 2018). Consequently, unlocking the dynamics of the Earth’s first billion year may reveal crucial aspects of the evolution of our – and other – planets.
Given the sparse data from this eon, high-performance computational modelling of the young Earth helps unravelling the dynamics of craton formation and the emergence of the first continents. Recent work (Capitanio et al., 2020) has shown a potential mechanism to explain the formation of the early continental cores in such a troubled young Earth – growing and stabilizing until maturity – and inherently illustrates a path for the tectonic evolution to present-day. Such models of the early Earth need to adopt the extreme conditions (a still hotter core and higher radiogenic heat production) that define a much more vigorous convection regime, consistent with the inferred 130-260 K higher potential temperatures in the Hadean-Archean mantle compared to today (Herzberg et al., 2010).
In addition, one must consider how such high temperature affects melting and dehydration of the mantle. Melting tends to weaken small parcels of rock, but melt extraction likely also impacts the large mass of the residual mantle. At present-day, the small degree of melting (<10%) in the ~8 km-thick basaltic oceanic crust implies an equivalent ~72 km thick layer of depleted mantle beneath, that is dehydrated and stiffer. In the hotter Archean Earth, melting degrees were higher (≤45%) and the segregated crust was thicker (30-50 km), which implies an up to 250 km thick mantle layer undergoing thermochemical differentiation through melt extraction, dehydration and stiffening (Herzberg et al., 2010).
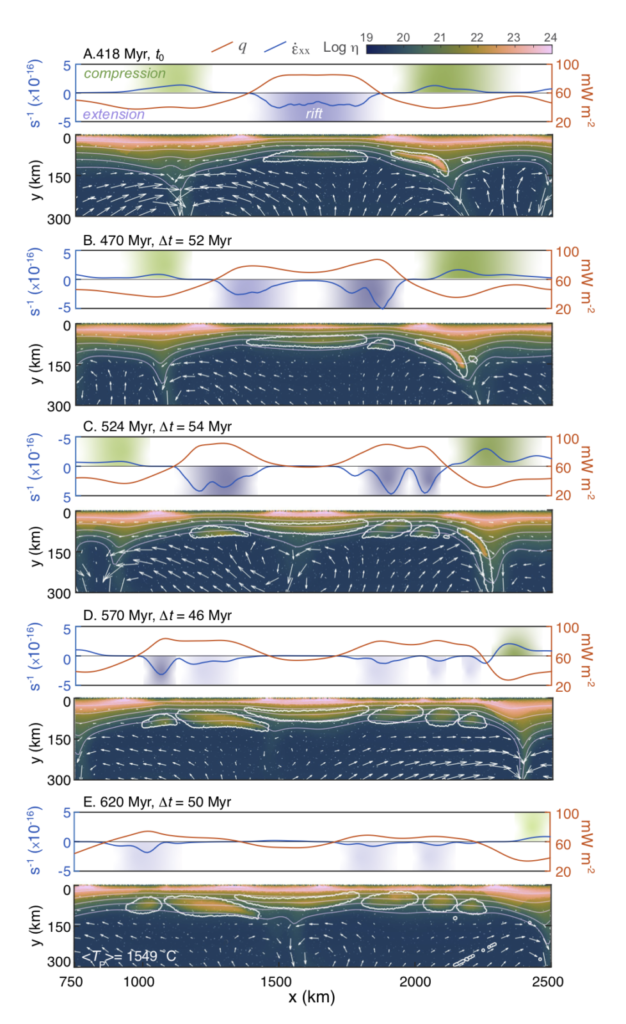
A numerical model of the early Earth tectonics over 200 Myr: Shown are viscosity fields with velocity vectors, surface heat flow and surface strain rates: (a) Decompression melting, dehydration and stiffening occur beneath the stretching lithosphere with the emplacement of a wide block with increased viscosity (white contour). (b) Within Δt ~ 52 Myr, rifting has ceased here and migrated laterally (purple shading in the strain rates), while thickening (green) occurs elsewhere. A new block of depleted CLM forms while the previous block beneath the original rift is cooling, as indicated by decreasing heat flow. (c-e) This process repeats with the lateral migration of rifting at intervals of ~50 Myr, with long-term formation of large volumes of depleted, stiff lithospheric blocks, and subsequent cooling. Contour lines for non-adiabatic temperatures T ≤ 1,300 °C are given every 260 °C, and the mantle potential time-averaged temperature ⟨TP⟩ is indicated [from Capitanio et al., 2020, Figure 1].
Such a scenario remains quite hectic until stabilization, with large melting and rapid recycling occurring throughout the first ~500 million years. Although lighter and more rigid, the initial blocks may in fact not be wide enough to resist convection stresses and large amounts of the initial lithosphere may be recycled into the mantle. This outcome provides a context for the disappearance of the Hadean crust. Zircons found on Earth today preserve traces of this and their chemical signature suggests crustal formation processes active as early as 4.2 billion years ago (Harrison et al., 2005). However, their traces embedded in the stable Archean lithosphere (<4 billion years old) give evidence for massive, likely complete recycling during the Hadean, just as suggested by modelling.
While these insights boost our understanding of early Earth’s tectonics, gaps in our knowledge obviously remain. One key aspect is the role of the yield strength of the lithosphere. A lower strength is known to favour plate formation and mobility, and their coupling with mantle convection (Moresi and Solomatov, 1998). On the contrary, thermochemical differentiation is maximised at lower yield strength, which thus counteracts lithospheric mobility via the mantle dehydration stiffening. In this process, formation of crustal rocks occurs in rift-like environments, while concurrent destruction and recycling occurs mostly via subduction-like margins (Figure 2). These highly mobile, plate tectonics-like features are also the site of large depletion, setting a negative feedback that rapidly suppresses lithosphere deformation by replacing it with dehydrated, stiffer lithosphere. Similar features are commonly found as fossil plate margin-like environments in many cratons. For instance, episodic subduction with arc-like signature, rifting with juvenile crust formation, and its reworking atop thinned lithosphere are documented in the Pilbara (van Kranendonk et al., 2007) and Kapvaal cratons (Simon et al., 2007).
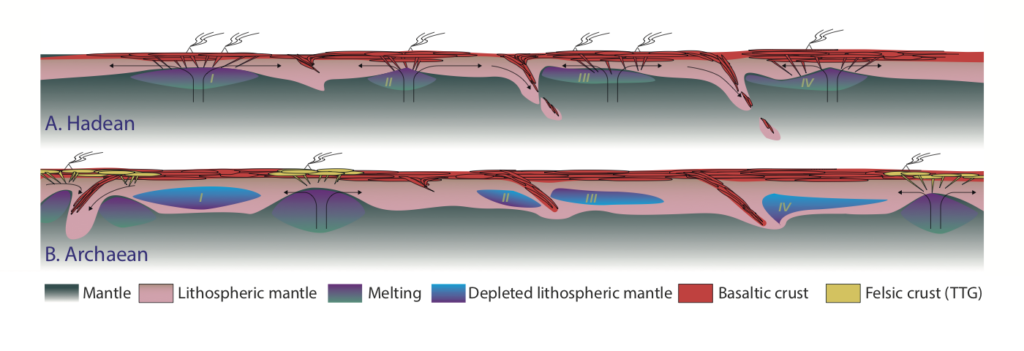
Concept of thermochemical lithosphere differentiation and stabilisation. (a) Likely Hadean conditions: proto-rifts and proto-convergent margins form and recycle primordial lithosphere (pink), basaltic crust (red), and large-scale mantle melting (green-purple). (b) Likely Archean conditions: Mantle stiffening due to melt extraction that progressively favours thickening and stabilisation of the lithosphere. Minor melting and downwellings allow intra-crustal melting and the differentiation of a felsic, TTG-like crust (yellow). [from Capitanio et al., 2020]
Further Reading
- Capitanio, F.A., Nebel, O. and Cawood, P.A. (2020), Thermochemical lithosphere differentiation and the origin of cratonic mantle, Nature, 588, 89-94.
- Harrison, T.M., Blichert-Toft, J., Müller, W., Albarede, F., Holden, P., Mojzsis, S.J. (2005), Heterogeneous Hadean hafnium: evidence of continental crust at 4.4 to 4.5 Ga, Science 310 (5756), 1947-1950.
- Herzberg, C., Condie, K. & Korenaga, J. (2010), Thermal history of the Earth and its petrological expression. Earth Planet. Sci. Lett. 292, 79–88.
- Korenaga, J. (2006), Archean geodynamics and the thermal evolution of Earth. In: Archean Geodynamics and Environments (eds Benn, K. et al.) 7–32 (American Geophysical Union).
- Van Kranendonk, M. J., Smithies, R. H., Hickman, A. H. & Champion, D. C. (2007), Secular tectonic evolution of Archean continental crust: interplay between horizontal and vertical processes in the formation of the Pilbara Craton, Australia. Terra Nova 19, 1–38.
- Lenardic, A. (2006), Continental growth and the Archean paradox. In: Archean Geodynamics and Environments (eds. Benn, K. et al.) 164–33 (American Geophysical Union).
- Lourenço, D.L., Rozel, A.B., Ballmer, M.D. and Tackley, P.J. (2020), Plutonic‐Squishy Lid: A New Global Tectonic Regime Generated by Intrusive Magmatism on Earth‐Like Planets, Geochemistry, Geophysics, Geosystems 21 (4), e2019GC008756.
- Moresi, L. & Solomatov, V. S. (1998), Mantle convection with a brittle lithosphere: thoughts on the global tectonic styles of the Earth and Venus. Geophys. J. Int. 133, 669–682.
- Rozel, A.B., Golabek, G.J., Jain, C., Tackley, P.J., Gerya, T. (2017), Continental crust formation on early Earth controlled by intrusive magmatism, Nature 545 (7654), 332-335.
- Simon, N. S. C., Carlson, R. W., Pearson, D. G. and Davis, G. R. (2007) The origin and evolution of the Kaapvaal cratonic lithospheric mantle. J. Petrol. 48, 589–625.