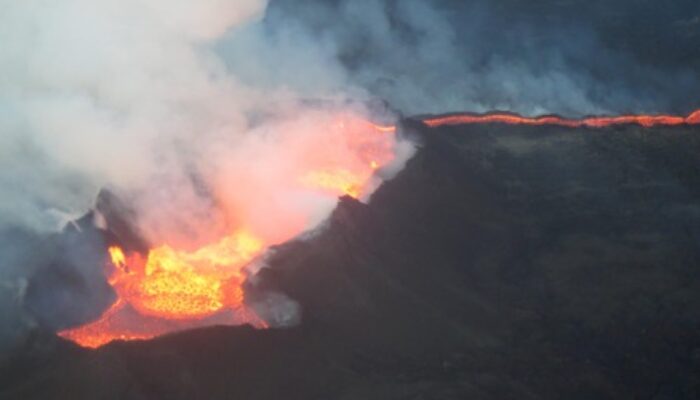
Across the Arctic lies evidence for a massive past volcanic event, called the High Arctic Large Igneous Province (HALIP). This ancient volcanic event is particularly unusual in that it appears to have lasted for over 50 million years. Could a single deep-seated mantle plume cause such wide-spread and long-lived volcanism? In a recent study published in the journal G-cubed (Heyn et al., 2024), we used numerical models of mantle convection to show that a rising mantle plume can indeed cause prolonged volcanic activity in the same area of a migrating plate if it interacts with changes in lithosphere thickness. In case of the Arctic, a plume that migrated from underneath the edge of the Arctic Ocean towards the thicker lithosphere of Greenland would trigger volcanism in the basin for at least about 40 Myr or more. Moreover, volcanic activity would happen in pulses rather than continuously, just as observed for HALIP.
The High Arctic Large Igneous Province (HALIP) is a massive volcanic province that erupted between ~130 to 80 Ma over large portions of the Arctic including Greenland, Canada, Svalbard and parts of Siberia (Figure 1). Today it is dispersed around the Arctic due to more recent tectonic events such as the opening of the Eurasian Basin. Apart from being wide-spread and long-lived, HALIP magmatism shows clear signs of pulses, with three peak phases previously identified at around 122 Ma, 95 Ma and 81 Ma (Dockman er al., 2018).
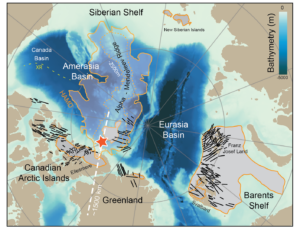
Figure 1: Map of today’s Arctic with the localities of HALIP marked by black lines (for mapped or inferred dykes) and orange regions (for inferred magmatic provinces beyond dykes). The red star marks the suggested position of the plume head arrival, and the white line indicates the selected profile for the numerical models. From Heyn et al. (2024).
Upwellings of mantle material are referred to as mantle plumes, and are responsible for many massive volcanic events around the world. Hawaii and Iceland are two modern examples of volcanic regions overlying a mantle plume. However, the particular combination of long-lived but periodic magmatism as observed for HALIP is difficult to reconcile with the classic view of plume-related melting beneath a moving plate, and therefore makes HALIP an especially interesting case.
Apart from the enigmatic HALIP, the Arctic is characterized by a vivid tectonic history including rifting, oceanic basin opening and closure, and collisional mountain ranges, which impart large changes in lithosphere thickness. In our study, we use numerical models of mantle convection to investigate how this structural inheritance may have influenced and shaped HALIP volcanism. For the first time as applied to the Arctic region, these models include processes of melting and melt migration, which require high resolution but capture more realistic feedback between melt and plume dynamics. The modeling was done using the open-source code ASPECT (Kronbichler et al., 2012; https://aspect.geodynamics.org). Our results reveal interesting behavior: a single plume can trigger pulses of volcanic activity in the same region without having internal pulsations of material flux, and later stages of melting happen far away from the plume (i.e. not directly above the plume conduit). Surprisingly, these pulses happen without changes in tectonic regime or external triggers.
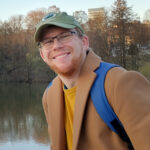
Björn Heyn is a postdoctoral researcher at the Centre for Planetary Habitability (PHAB) at the University of Oslo, Norway. He is interested in different aspects of mantle plumes, from their formation at the core-mantle boundary to their interaction with the lithosphere at the Earth’s surface. Currently, he is part of the POLARIS project on the Evolution of the Arctic in Deep Time, funded by the Norwegian Research Council Young Talent Grant 326238.
Interaction between the lithosphere and the plume
Since the aim of this study was to understand the first-order plume-lithosphere dynamics behind HALIP, several simplifications were made for these 2-D models. One of them concerned the inherited lithospheric structure of the Arctic, which is implemented by different configurations of a gradual or a step-wise increase of the lithosphere thickness from north to south, representing the transition from an oceanic Basin (Amerasia Basin) to a continental margin (Sverdrup Basin) to a craton (Greenland) as shown in Figure 2a.
A rising mantle plume with a mushroom-like head hits underneath the basin, but (depending on the setup) subsequently interacts with the changes in lithosphere thickness as the plate moves relatively northwards over the plume. After an initial stage of melting in and above the plume head at (a classic large-igneous-province-forming event), the model eventually reaches a melt-free stage (at ~28-40 Myr model run time). Afterwards, at around 40-48 Myr, a short period of rejuvenated melting occurs. Interestingly, rejuvenated melting happens in the same region of the basin where the plume head originally hit the lithosphere (Figure 2c). At this time, the plume, now resembling a stem-like channel, is located further south underneath the craton. Melting occurs because plume material is channeled along the base of the lithosphere towards the basin, where it triggers local small-scale convection underneath the recently thinned lithosphere.
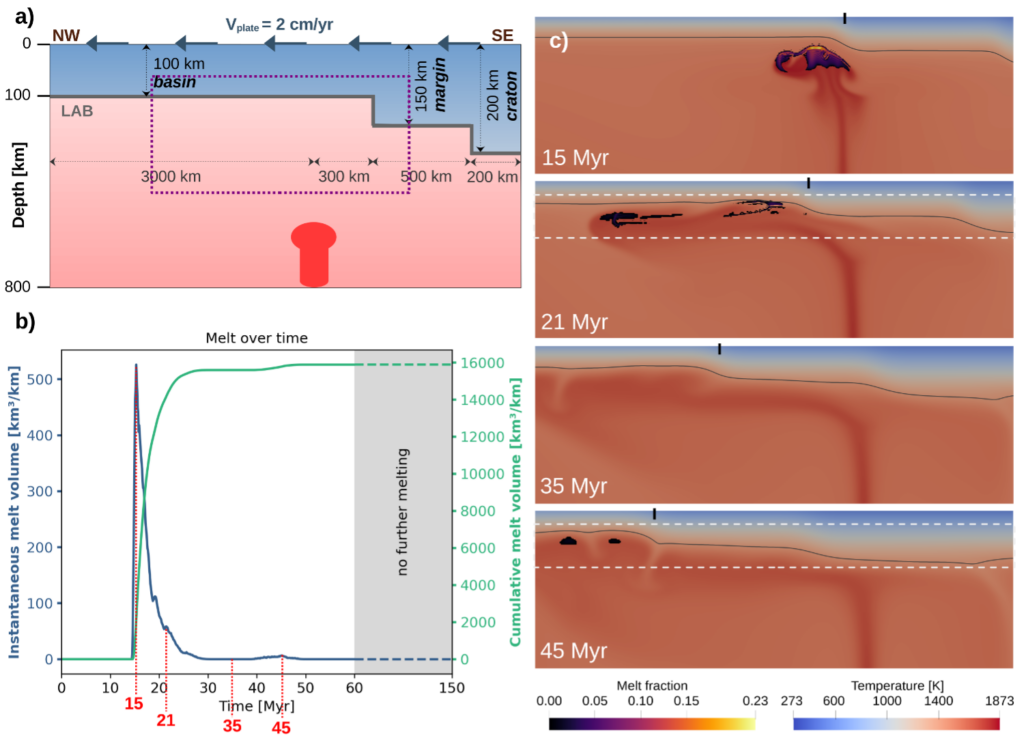
Figure 2: The model setup (a), the calculated melt over time (b), and snapshots of the temperature and the melt at specific times (c). Taken from Heyn et al. (2024).
Since the plume stem is much weaker than the earlier plume head, rejuvenated melting produces much smaller volumes. The recovered volumes of this later event are strongly dependent on two aspects: (1) the dynamic feedback between melt and the mantle, which facilitates strong local thinning and the formation of undulations in lithosphere depth during the first melting event, and (2) the presence of a (pre-existing) change in lithosphere thickness that channels the plume towards these regions. A scenario run with no relative motion between plume and plate predicted a prolonged melting period of at least 40 Myr with temporal and spatial variations in magmatic volumes, but no separated pulses. In contrast, if the steps in lithosphere thickness are 500km of more away from the initial plume position, magmatic pulses are absent regardless of the plate motion.
Reconciling observations and models of HALIP
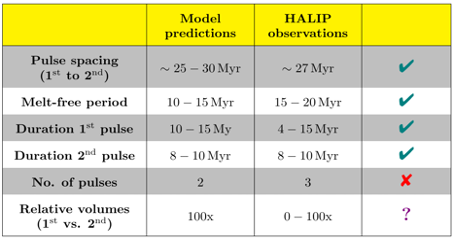
Figure 3: Comparison of model predictions and observations of HALIP from the study of Heyn et al. (2024).
Patterns of magmatic activity predicted by these models can explain several aspects of HALIP: two out of three pulses can be reproduced, with similar duration and timing as observed in the Arctic (see Figure 3). Despite the models failing to reproduce a third pulse, the study clearly shows that a plume can cause prolonged, multi-phase magmatism in settings applicable to the Arctic. Changes in tectonic regime (such as rifting) or pulses in plume flux (for example from slab-plume interactions at the base of the mantle) could further modify the magmatism, but are not necessary to produce complex magmatic patterns in the presence of lithosphere thickness changes. Finally, a key take home message is that plume-related magmatism does not directly mark the position of the plume, since the last phase of HALIP magmatism most likely happened when the plume was beneath the Greenland craton, more than 500 km away from the eruptions.
You can check the full publication here:
Heyn, Björn H., Shephard, Grace E., and Conrad, Clinton P. (2024). Prolonged multi-phase magmatism due to plume-lithosphere interaction as applied to the High Arctic Large Igneous Province, Geochemistry, Geophysics, Geosystems, 25, e2023GC011380, doi: 10.1029/2023GC011380, https://agupubs.onlinelibrary.wiley.com/doi/10.1029/2023GC011380
To know more and follow updates on the POLARIS project:
https://polarisdigital.org
https://prosjektbanken.forskningsradet.no/en/project/FORISS/326238
Instagram: @polarisdigitalarctic
X: @ShepGracie and @PolarisArctic
References
Dockman, D., Pearson, D., Heaman, L., Gibson, S., and Sarkar, C. (2018). Timing and origin of magmatism in the Sverdrup Basin, northern Canada - implications for lithospheric evolution in the High Arctic Large Igneous Province (HALIP), Tectonophysics, 742-743 , 50–65, doi: 10.1016/j.tecto.2018.05.010
Kronbichler, M., Heister, T., and Bangerth, W. (2012). High accuracy mantle convection simulation through modern numerical methods, Geophysical Journal International, 191 , 12–29, doi: 10.1111/j.1365-246X.2012.05609.x