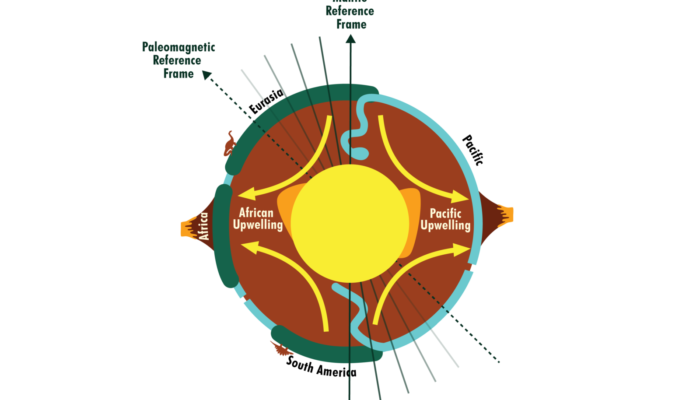
Have you ever pondered the significance of reference frames in the reconstruction of tectonic paleo positions? In this edition of News & Views, we feature Jonathon Leonard as he delves into this subject, aided by illustrations from Rachel O’Brien.
In the 1600s, in an effort to show why we cannot feel the Earth orbiting the sun at speed, Galileo came up with a thought experiment. In his experiment, a person sits in a ship’s cabin with no windows and hangs up a bottle that drops water from the ceiling. Galileo says that the water dripping would be no different whether the ship was at rest or in constant motion. This was an early declaration of inertial frames of reference. The concept of a frame of reference is where motions must be described in relation to another point of reference and is still relevant today. One surprising application is in plate tectonics. When positioning past tectonic plates as they move over the surface of the Earth, we need to consider the reference frame in which these motions are measured against. We can reconstruct plate motion relative to the spin-axis of the past Earth but also define it relative to the mantle. Which frame to use and how to calculate it is not straightforward. In this blog, we will briefly summarize how tectonic plate positions are reconstructed, how reference frames are derived, and some differences that emerge from different reference frames.
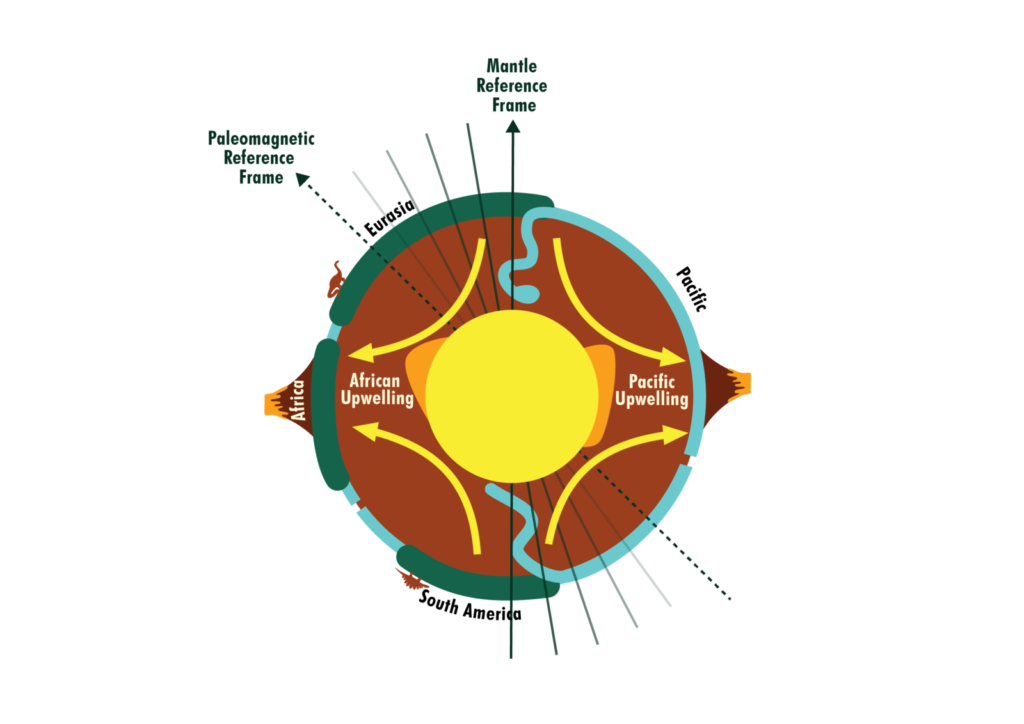
Mantle reference frames use mantle features such as upwellings and subducted slabs as an absolute reference to plate motion. These reference frames do not always reflect the true position of past continents as they neglect true polar wander. This is shown in the figure with the implied spin axis of the Earth in a mantle frame actually in a different place in the paleomagnetic frame due to a shifting of the plate-mantle system relative to the core. Image by Rachel O’Brien and based on Conrad et al, 2013.
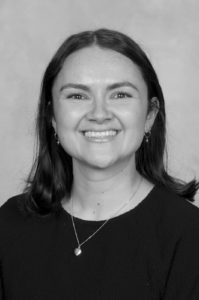
Rachel O’Brien
Visual Arts Teacher at Brigidine College St Ives
Freelance Illustrator at Studio Mallee
Reconstructing where plates were in the past.
Ever since the earliest world maps were constructed, rudimentary plate ‘reconstructions’ were done by fitting the continents back together based on their shape. Alfred Wegener added more evidence, such as correlations between fossils and glacial deposits. Until recently, most reconstructions were done similarly, where estimates of the location of continents and ocean basins were based on data such as ocean paleomagnetic surveys, sedimentary deposits, etc. (see Seton et al. 2023 for a great review of plate reconstructions). As methods got more sophisticated, we eventually got to a model where we could continuously and consistently reconstruct the entire jigsaw of plates through time using software such as GPlates. The most effective way to prescribe plate motion is to have it move relative to another plate. Because these plates are on the surface of a sphere (sort of), the easiest way to describe that movement is as a rotation around some imaginary axis going through the Earth (look up Euler rotations for more detail). To ensure all the plates move consistently, we need to have all plates moving relative to one another. For example, a small plate such as Sibumasu in South-East Asia might move as a rotation relative to Indochina, which moves relative to Eurasia, which then moves relative to Africa. This happens for all plates and thus builds a reconstruction tree where all plates move relative to each other until they reach one plate at the top of the hierarchy (often, this is Africa).
At this point, we have a whole system of plate motions; however, we only know relative plate motions, and we don’t know where the plates actually were in the past. To find the absolute location of the plate system, we need to add another rotation at the top of the hierarchy that rotates the plates into an absolute reference frame. This is where we have a conundrum. The absolute motion of plates can be defined relative to the mantle or the magnetic poles. Both result in similar reconstructions with some important differences.
Absolute reference frames: mantle vs paleomagnetic
In an ideal world, we could reconstruct plates back to the exact latitude and longitude of the past. We can get some of this information using magnetic minerals in the rock aligning to the Earth’s magnetic upon their creation. The dip of the mineral relative to the horizontal is proportional to the direction of the Earth’s magnetic field and, therefore, proportional to the paleo-latitude of the mineral when it was created (assuming the magnetic field aligns with the spin axis of the Earth on average over long time spans). Collating enough paleomagnetic data allows us to construct an absolute reference frame called a paleomagnetic reference frame. Unfortunately, while this paleomagnetic reference frames can record paleo-latitude, the paleo-longitude is not recorded, leading to a bit of guesswork when working out how far east or west plates were in the past. There are also issues with errors, compaction of rocks, etc., that limit the reliability of a paleomagnetic frame.
Alternatively, mantle reference frames can be used. These record plate motions relative to the mantle. We can do this by assuming features of the mantle to be more-or-less persistent back through time. For example, seamount chains such as the Hawaii-Emperor chain can be interpreted as surface manifestations of long-lived hotspots within the mantle. We can also use tomography to identify subducted ocean slabs and ‘rewind’ it to track where plate boundaries may have been in the past. These methods are pretty effective at constraining plate motions with the caveat that these motions are viewed from the standpoint of the mantle. While this is perfectly fine for use in things like geodynamics, the mantle frame has some downfalls, most notably that it neglects True Polar Wander.
Should I be worried about True Polar Wander?
The mantle frame is often the ‘default’ reference frame in plate tectonic reconstructions; for example, it is the reference frame that comes with the included data in a GPlates download. However, because the mantle frame has the mantle as the frame of reference, it only records the movement of the plate relative to it. It neglects any movement of the whole plate-mantle system relative to the core. This shift occurs due to changes in the mass distribution of the mantle and is called True Polar Wander. The effect of True Polar Wander is significant on long-time scales and can result in differences of up to 15 degrees in latitude. When it comes to things like understanding paleoclimates and things related to that, like the evolution of life, the true latitude of the Earth matters as it is directly related to things like temperature, wind direction, etc. As paleomagnetic reference frames record the true paleo-latitude, a paleomagnetic reference frame should be preferred. On the other hand, a mantle frame is perfectly suitable for studies that only consider the surface of the Earth relative to the mantle, such as mantle convection modelling.
Last month, we presented the results of climate simulations done over the previous 250 million years with a mantle and paleomagnetic reference frame. We found that different reference frames can result in different average global temperatures of up to 1 degree Celsius at certain times. More striking, we found that the exact location in different reference frames could differ by over 15 degrees latitude and have average annual temperatures that vary by over 20 °C. These differences tend to be around mid-latitudes, where a slight change in latitude often results in very different wind directions. This research demonstrates that the concept of reference frames, as thought up by Galileo centuries ago, is relevant today and has important implications for how we understand the past climate.
References and bedtime reading
- Conrad, Clinton P., Bernhard Steinberger, and Trond H. Torsvik. “Stability of Active Mantle Upwelling Revealed by Net Characteristics of Plate Tectonics.” Nature 498, no. 7455 (June 2013): 479–82. https://doi.org/10.1038/nature12203.
- Cox, Allan, and R. B. Hart. Plate Tectonics: How It Works. John Wiley & Sons, 1991.
- Leonard, Jonathon, Sabin Zahirovic, Tristan Salles, and Claire Mallard. “Exploring the Role of Mantle and Paleomagnetic Reference Frames with Intermediate Complexity Climate Models.” Copernicus Meetings, February 22, 2023. https://doi.org/10.5194/egusphere-egu23-10673.
- Müller, R. Dietmar, John Cannon, Xiaodong Qin, Robin J. Watson, Michael Gurnis, Simon Williams, Tobias Pfaffelmoser, Maria Seton, Samuel H.J. Russell, and Sabin Zahirovic. “GPlates: Building a Virtual Earth Through Deep Time.” Geochemistry, Geophysics, Geosystems 19, no. 7 (July 1, 2018): 2243–61. https://doi.org/10.1029/2018GC007584.
- Seton, Maria, Simon E. Williams, Mathew Domeier, Alan S. Collins, and Karin Sigloch. “Deconstructing Plate Tectonic Reconstructions.” Nature Reviews Earth & Environment 4, no. 3 (March 2023): 185–204. https://doi.org/10.1038/s43017-022-00384-8.
- Torsvik, Trond H., Rob van der Voo, Pavel V. Doubrovine, Kevin Burke, Bernhard Steinberger, Lewis D. Ashwal, Reidar G. Trønnes, Susan J. Webb, and Abigail L. Bull. “Deep Mantle Structure as a Reference Frame for Movements in and on the Earth.” Proceedings of the National Academy of Sciences 111, no. 24 (June 17, 2014): 8735–40. https://doi.org/10.1073/pnas.1318135111.
- van Hinsbergen, Douwe J. J., Lennart V. de Groot, Sebastiaan J. van Schaik, Wim Spakman, Peter K. Bijl, Appy Sluijs, Cor G. Langereis, and Henk Brinkhuis. “A Paleolatitude Calculator for Paleoclimate Studies.” PLOS ONE 10, no. 6 (June 10, 2015): e0126946. https://doi.org/10.1371/journal.pone.0126946.
Xianwu Xin
The continents are constantly “westdrift“, as Wegener put it.
During the drift process, the shape and velocity of the continent-mass will constantly change.
The harmonic motion of the Earth is described using five reference frames. Their datums are the Earth’s core, mantle, New Zealand, no net rotation, and Greenwich. These datums can be converted each other. See the attachment of EGU23-122. It is currently unclear how much deviation there is between the results of geomagnetic research and the calculation results of harmonic motion. In Figure 3B, the figures in italics below the latitude line are the calculated values of drift velocity of each the continent-mass relative to the New Zealand datum. Building a paleomagnetic reference frame using the New Zealand benchmark may be more convenient.
Please correct any inaccuracies. Thanks!