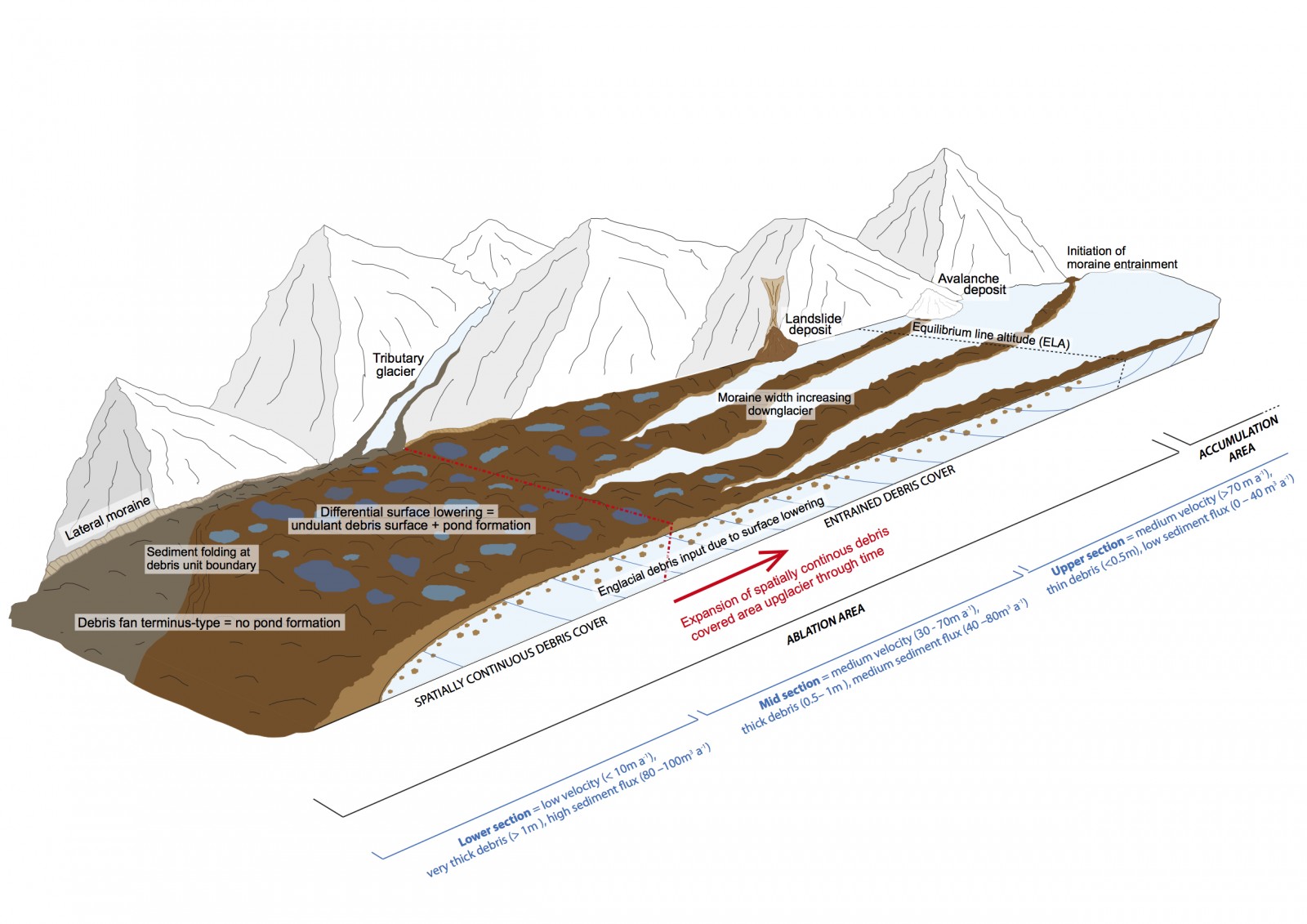
There is still a huge amount we don’t know about how glaciers respond to climate change. One of the most challenging areas is determining the response of debris-covered glaciers. Previously, we have reported on a number of fieldwork expeditions to debris-covered glaciers but with this Image of The Week we want to show you another way to investigate these complex glaciers – numerical modelling!
Debris-covered glaciers
Debris-covered glaciers occur globally, with a great many being found in the Himalaya-Karakoram mountain range. For example, in the Everest Region of Nepal 33% of glacier area is debris covered (Thakuri et al., 2014). The response of debris-covered glaciers to future climate change in such regions has huge implications for water resources, with one fifth of the world’s population relying on water from the Himalayan region for their survival (Immerzeel et al., 2010).
Debris-covered glaciers respond to climate change differently to debris-free glaciers as the supraglacial debris layer acts as a barrier between the atmosphere and glacier (Reznichenko et al., 2010). The supraglacial debris layer has several key influences on the glacier dynamics:
- Glacier ablation (loss of mass from the ice surface) is enhanced or inhibited depending on debris layer thickness and properties – see our previous post.
- Supraglacial debris causes glaciers to reduce in volume through surface lowering rather than terminus retreat (typical of debris free mountain glaciers).
Understanding the influence of a supraglacial debris layer on mass loss or gain is, therefore, key in determining the future of these glaciers. The properties of supraglacial debris layers can vary in time and space both in debris layer thickness and distribution, as well as properties of the rocks which make up the debris (e.g. albedo, surface roughness, porosity, size and moisture content). It is these characteristics of the debris-cover which control the heat transfer through the debris and therefore the amount of thermal energy that reaches the underlying ice causing melting (Nicholson and Benn, 2006). In order to better predict the future of debris-covered glaciers we needs to be able to numerically model their behaviour. This means we need a better understanding of the variations in debris cover and how this affects the ice dynamics.
How does a supraglacial debris layer vary in time and space?
Our Image of the Week (Fig. 1) shows a schematic of how debris distribution can vary spatially across a glacier surface and also this can change through time. The main inputs of debris are:
- Upper regions: snow and ice avalanches in the upper reaches of the glacier.
- Mid and Lower regions: rock avalanches and rock falls (Mihalcea et al., 2006).
These irregular mass movement events vary in frequency and magnitude, and therefore affect debris distribution across the glacier surface but also through time. The irregularity of them makes it really hard to predict and simulate! Luckily, debris transport is a little more predictable.
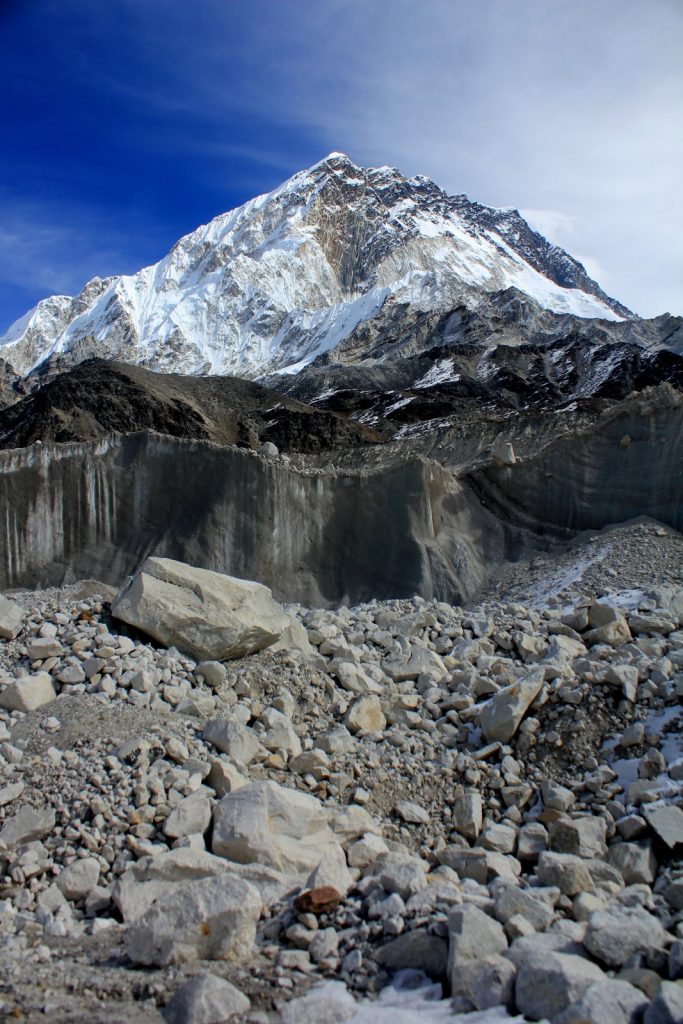
Figure 2: An ice cliff emerging out of the supraglacial debris layer on Khumbu Glacier, Nepal, with Nuptse in the background. [Credit: M. Gibson]
Debris is initially transported along medial moraines (glacially transported debris) in the upper and mid-sections of the glacier, this is known as entrained debris. The various sources of entrained debris combine to form a continuous debris cover in the lower reaches of the glacier (Fig. 1). As a supraglacial debris layer is forming, such as for Baltoro glacier (Fig. 1), the boundary between the continuous debris layer and entrained debris sections progresses further upglacier over time.
Eventually transported debris will reach the terminus of the glacier and be deposited (Fig. 1), mainly due to a decrease in surface velocity of the glacier towards the terminus. However, once debris is deposited it doesn’t just sit there; debris is constantly being shifted around as ablation (surface melting) occurs. As ablation occurs the debris surface ablates unevenly, as the thickness of the debris layer is spatially variable. Uneven ablation, otherwise known as differential surface lowering, causes the glacier surface to be made up of topographic highs and lows, the latter of which sometimes become filled with water, forming supraglacial ponds (Fig. 1) . Another product of debris shifting is that ice cliffs, such as the one seen in Fig. 2, are exposed. These features are initially formed when englacial channels collapse or debris layers slide (Kirkbride, 1993). All this movement and shifting means that not only do glacier models have to consider variation in debris layers across the glacier and through time, but also the presence of ice cliffs and supraglacial ponds. They are important as they have a very different surface energy balance to debris-covered ice. To complicate things further the frequency and area of ice cliffs and supraglacial ponds also vary through time! You see the complexity of the problem…
Modelling spatially and temporally varying debris layers
Numerical modelling is key to understanding how supraglacial debris layers affect glacier mass balance. However, current numerical modelling often either omits the presence of a supraglacial debris layer entirely, or a debris layer that is static in time and/or space (e.g. Collier et al., 2013; Rowan et al., 2015; Shea et al., 2014). However, as outlined earlier, these supraglacial debris layers are not static in time or space. Understanding the extent to which spatiotemporal variations in supraglacial debris distribution occur could aid identification of when glaciers became debris-covered, glaciers that will become debris-covered glaciers in the future, and the timescales over which supraglacial debris layers vary. The latter is particularly relevant to numerical modelling as it would result in total glacier ablation being calculated more precisely throughout the modelling time period. Understanding the interaction between glacier dynamics and debris distribution is therefore key to reconstructing debris-covered glacier systems as accurately as possible.
Edited by Emma Smith
Morgan Gibson is a PhD student at Aberystwyth University, UK, and is researching the role of supraglacial debris in ablation of Himalaya-Karakoram debris-covered glaciers. Morgan’s work focuses on: the extent to which supraglacial debris properties vary spatially; how glacier dynamics control supraglacial debris distribution; and the importance of spatial and temporal variations in debris properties on ablation of Himalaya-Karakoram debris-covered glaciers. Morgan tweets at @morgan_gibson, contact email address: mog2@aber.ac.uk.
Pingback: Cryospheric Sciences | Image of the Week – Making waves: assessing supraglacial water storage for debris-covered glaciers
Pingback: Cryospheric Sciences | Image of the Week – Climbing Everest and highlighting science in the mountains
Pingback: Cryospheric Sciences | Image of the Week – We walked the Talk to Everest
Pingback: Cryospheric Sciences | Image of the Week – When “Ice, Ice Baby” puts rocks “Under Pressure”
Pingback: Cryospheric Sciences | Image of the Week – Looking to the past for answers
Pingback: Cryospheric Sciences | Climate Change and Cryosphere – What can we learn from the smallest, most vulnerable glaciers in the Ötztal Alps?