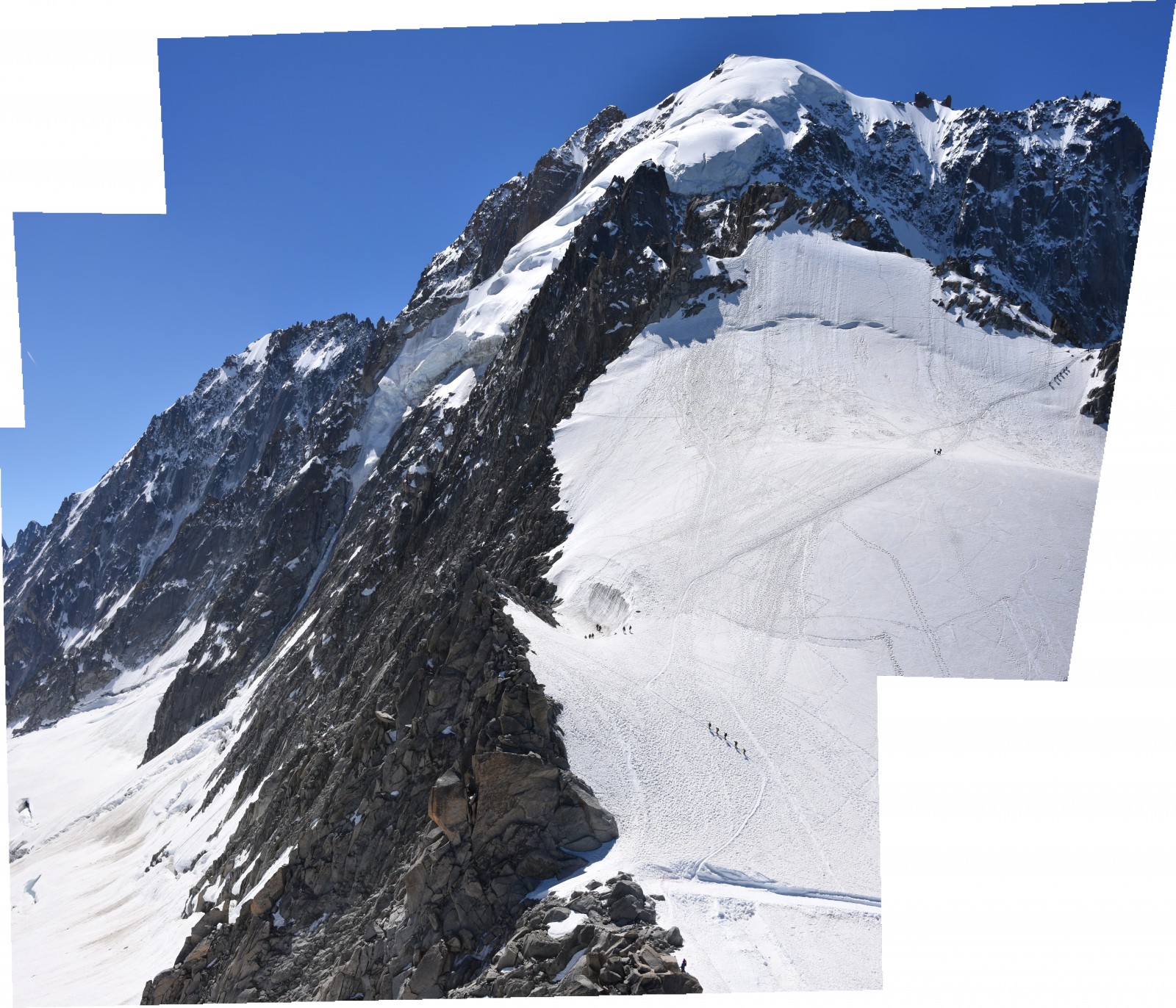
Image 1: Composite image of the Aiguille Verte, the heavily-fractured headwall of the Glacier d’Argentière near Chamonix, France [Credit: D. Dennis].
Bowie and Queen said it first, and Vanilla Ice brought it back. But now, I’ve set out to quantify it: Pressure. Rocks in glacial landscapes can experience many different kinds of pressure (forces), from sources like regional tectonics or even the weight of the glacier itself. Our hypothesis is that smaller-scale pressures, caused by the formation of ice in small bedrock cracks (frost-weathering), have a large effect on the sculpting of landscapes in cold regions. This post will share how we evaluate these processes and their dependence on temperature, as well as discussing the broader effects for glacier and glacial landscape evolution.
Walking through the valley in the shadow of glaciers
Growing up just outside Glacier National Park, USA, at nearly the exact edge of the former Laurentide Ice Sheet, I became familiar with the romantic lore of how we understand glacial landscapes (Images 2, 3). Observing these glacial landscapes later throughout my formal Earth science education, I came to understand mountains as passive resistors to the relentless efficiency of glacier advance, erosion, and retreat—offering evidence of past glaciations but nonetheless devoid of agency in the rise and fall of icy stadials.
My current PhD research, however, investigates a slightly-modified premise: that glaciers and their landscapes respond in concert with climate, and that dividing the dynamics governing the ice and the rock may not be as straightforward as once thought. My work is a sub-project of the Climate Sensitivity of Glacial Landscape Dynamics (COLD) project, funded by the European Research Council (ERC) and lead by Dirk Scherler at the Deutches GeoForschungsZentrum (GFZ) in Potsdam, Germany.
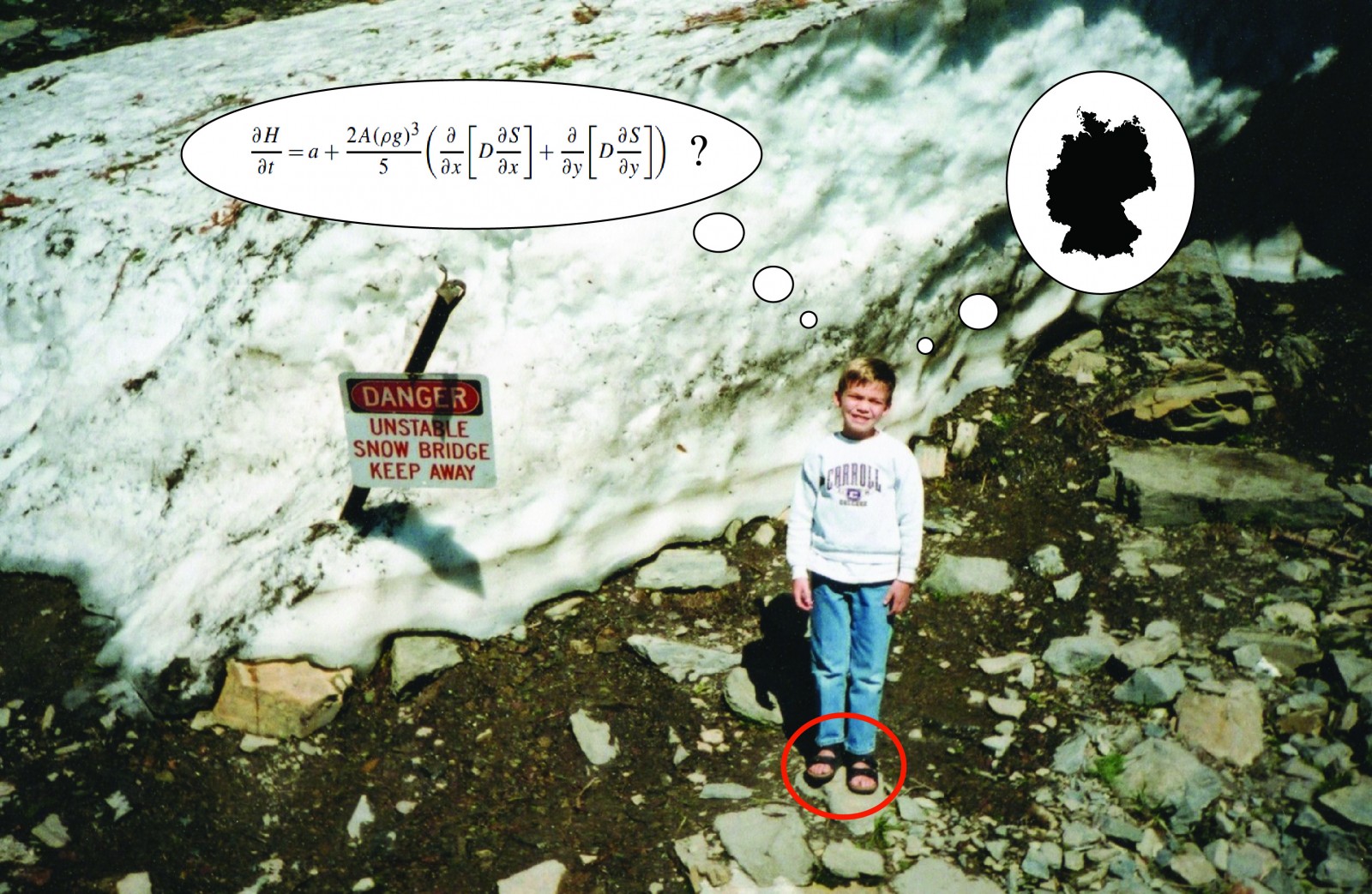
Image 2: The author on holiday in Glacier National Park, Montana, circa 2001, demonstrating an early aptitude for glacial geomorphology and cosmogenic nuclide geochemistry. His affinity for popular German footwear at a young age foreshadowed his eventual move to Germany to study glaciology and geomorphology [Credit: D. Dennis].
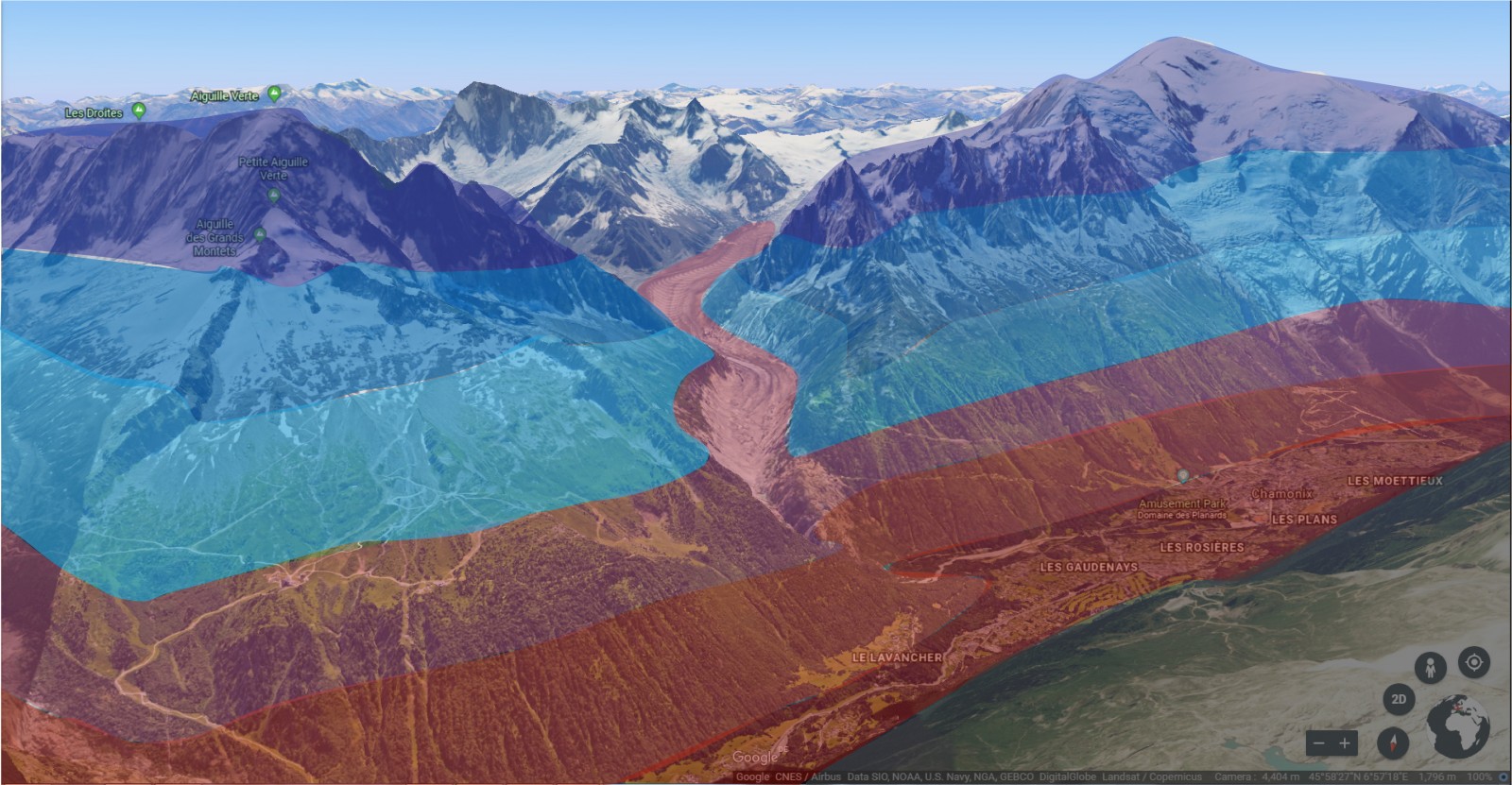
Image 3: This image of Chamonix Valley and the M. Blanc massif conceptually outlines how average annual temperature may change with elevation in steep hillslopes. The highest peaks in the massif tower up to nearly 4000 m over Chamonix Valley, which sits at appx.1000m. This corresponds to a nearly ~20 °C difference in annual average temperature. [Image adapted from Google Earth].
Temperature as a control in glacial landscapes
Glaciers exist in locations with temperatures that are, for some portion of the year, below freezing, as this is a condition required for snow to persist through the melt season and to form ice. Temperature is therefore an important primary control on the stability of glaciers. These cold temperatures, however, impact mountain environments beyond just the formation/decline of glaciers, and several decades of recent research have shown that temperature is an important controlling factor on the type and magnitude of erosion (the act of dislodging and transporting rock) in cold landscapes.
Mountain glacier valleys are commonly characterized by steep head- and sidewalls which frame the glacier within (like in our Image of the Week). At our field sites in the French, Swiss, and Italian Alps, these rockwalls can tower up to 1500 m above the surface of the glaciers, corresponding to a temperature gradient of ~10 degrees (Image 3). Therefore, the rocks at different elevations are exposed to different temperature conditions, which could lead to differences in the rate of erosion.
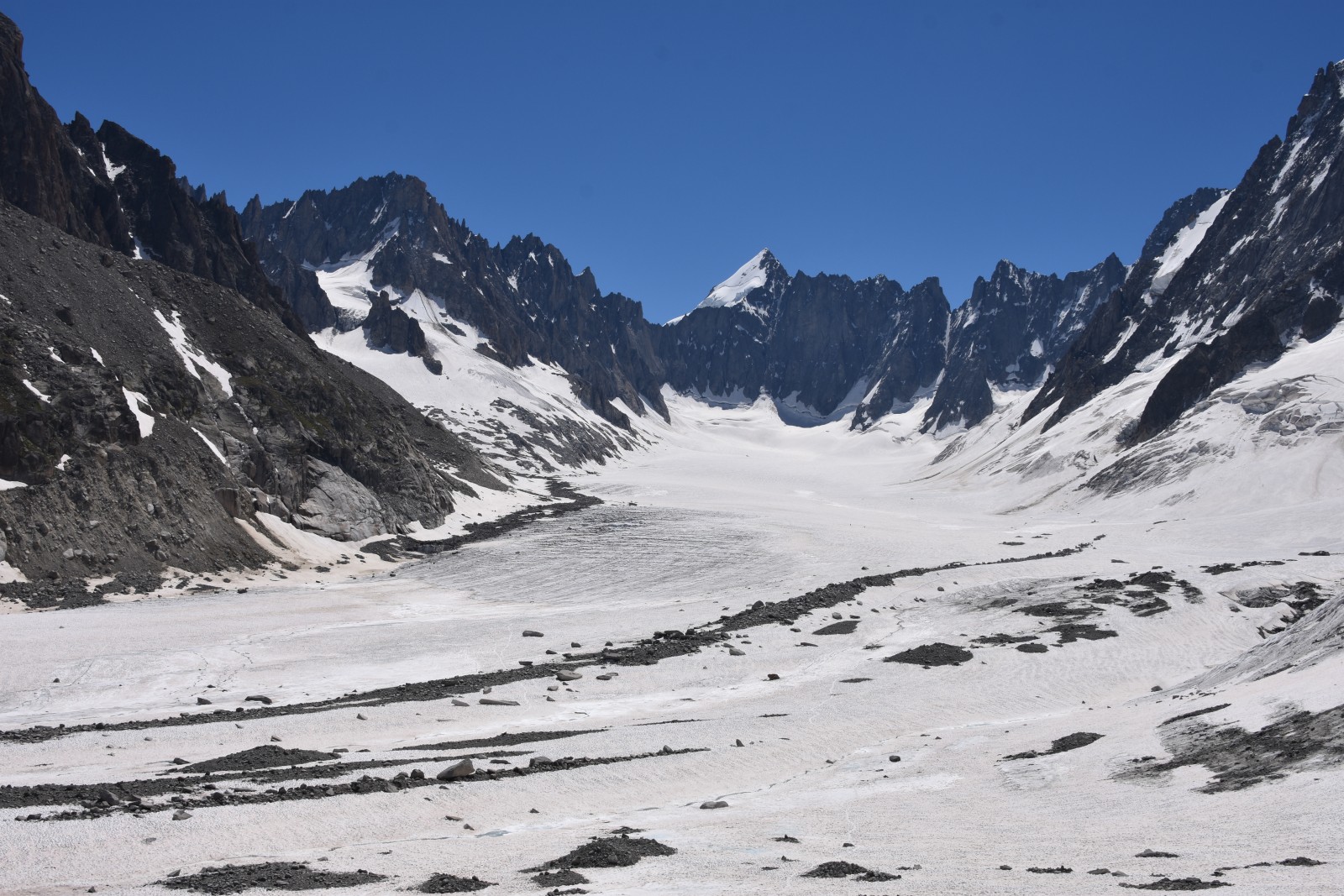
Image 4: Permafrost degradation and frost-weathering in the steep hillslopes of the M. Blanc massif commonly lead to the deposition of debris on the glaciers at the base of the mountains. Shown here is Glacier d’Argentière (France) with patches of surface debris [Credit: D. Dennis].
Erosion in steep rockwall faces
Frost-weathering processes occur only at temperatures at or below zero, therefore requiring the same cold temperature conditions that form glaciers. At these temperatures, liquid water present in small cracks in the bedrock freezes. The pressure exerted on the rock by the ice as it freezes causes the rock to fracture, leading to large cracks in the bedrock (Image 5). Erosion occurs when the ice in the crack becomes large enough and its corresponding fracture wide enough that the rock can no longer remain attached and it falls from the rockwall surface.
Erosion can also occur when the ice in the crack melts and no longer “cements” the surface together. Because temperatures in glacial landscapes are commonly quite cold, much of the bedrock is considered permafrost (permanently-frozen ground), and remains frozen throughout the year. In the Alps, however, warmer temperatures over the past decades have caused the permafrost to thaw, melting the lenses of ice and causing larger and more frequent rockfalls.
Temperature conditions are therefore important for both the rate at which cracks form in rocks (and erode from the surface) in addition to permafrost stability and the size/frequency of rockfalls. As temperatures change in mountain regions due to global warming, this could lead to considerable changes in debris production.
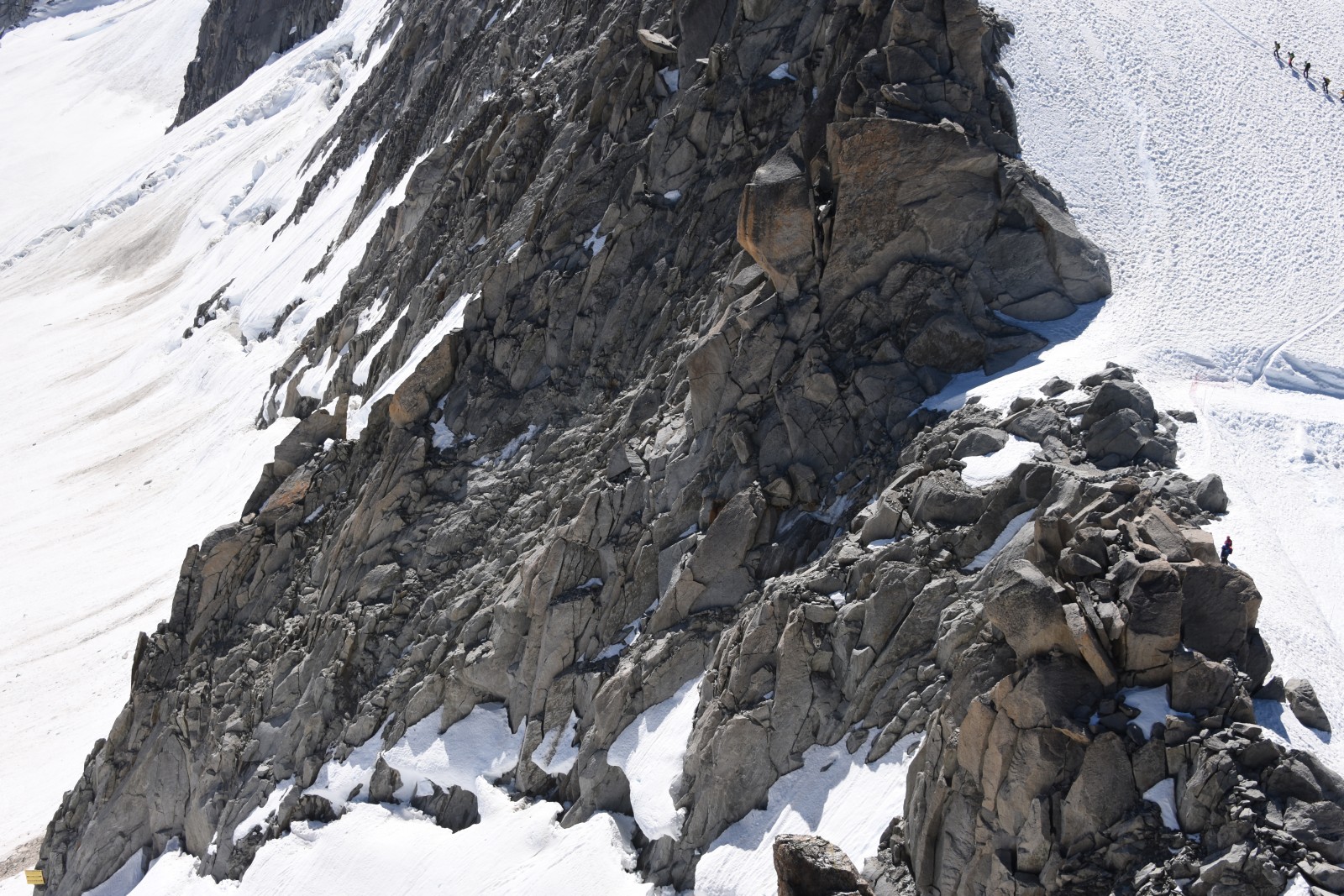
Image 5: A cropped version of our Image of the Week, showing the base of the Aiguille Verte, headwall of Glacier d’Argentière. Large fractures in the bedrock are clearly visible. These may have grown from much smaller cracks that formed due to frost-weathering.
The hillslope/glacier surface connection
After material erodes from the surface of the headwall, it is often deposited onto the surface of the glacier (Image 3). As mentioned above, the deposition of material can occur both at a constant rate or sporadically (as in the case of permafrost-thaw rockfalls), depending on the controlling process. As such, determining the actual representative rate at which these headwalls erode is challenging.
Though this work can be complicated, we believe it to be important, as debris deposited on the surface of glaciers can insulate the ice from the effects of temperature (Image 4, Video 1). Though the global distribution of debris-covered glaciers is much smaller than debris-free glaciers, debris-covered glaciers make up a non-trivial fraction of the glaciers in populated mountain regions where they may be important fresh water sources, contribute to glacial hazards, or allow for the generation of hydropower. Understanding the supply of debris to these glaciers (via erosion), and how it may change, is therefore an important component of forecasting their evolution under warming climates.
Video 1: This drone footage from the Arolla Glacier, Switzerland, shows the steep relief which can develop as a result of differential melting. Debris thicker than 2-4 cm insulate ice, leading to topographic relief on the glacier surface as exposed ice melts and covered ice is protected. [Credit: D. Gök, GFZ]
Re-evaluating the dynamic glacial landscape
Though studies of frost-cracking and debris-covered glaciers individually are not necessarily brand new inventions, our methods for combining the two are rather novel. In doing so, we are linking the evolution of glacier with the evolution of the landscape itself, and investigating an interesting feedback loop induced by changes in climate. Should erosion rates increase with warmer temperatures, and the mountains therefore supply more debris to glacier surfaces, this could extend the “lifetime” of the glacier by insulating it; likewise, if erosion rates decrease, less debris supplied to already-covered glaciers could lead to less insulation and (comparatively) higher melt rates. This interplay demonstrates the complexity of Earth system processes, and the need to take these complexities into account when considering the effects of climate changes.
To summarize
Pressure, pushing down on rock,
Pushing laterally against rock, can cause them to fall.
Under (thick) debris, glacier melt will slow,
Despite higher temperatures,
And global warming.
Will it ever stop? I don’t know.
Turn up the temperatures, then no more (ice and) snow.
At the end of the day, frost-weathering needs ice,
When water can’t freeze, ice-cracking’s no dice.
Edited by David Docquier
Donovan Dennis is a PhD student at the Deutches GeoForschungsZentrum in Potsdam, Germany. He is interested in many aspects of glaciology and glacial geomorphology, and currently investigates the geomorphic feedbacks on glacial landscape erosion. He previously worked on post-deposition alteration of stable water isotope signals in snow and ice. He tweets as @donovan__dennis.
Contact Email: dennis@gfz-potsdam.de