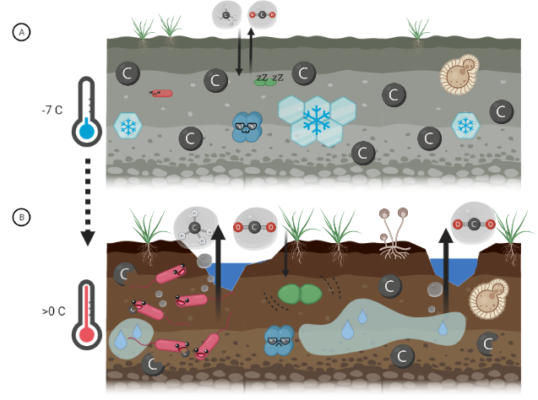
Did you know that the oldest organism on Earth is believed to be a microorganism found in 3-million-year-old permafrost in Siberia? There, it was living at a cosy average temperature of -10 °C at 14 m depth. Or did you hear that some other Arctic soil microorganisms can happily live at extreme temperatures down to -40 °C? Scientists often use these “extreme” microorganisms to get an idea on how extra-terrestrial life could look like. However, these microorganisms are also important on our planet as they are a crucial connection between the environment and climate. In this blog post, we’ll see how microbes, invisible to the bare eye, can survive in such cold environments and why their hunger for carbon matters during global warming.
(C)old permafrost as a habitat
Permafrost soils can be found at both poles and in mountain terrain, where temperatures are low enough for the soils to be frozen throughout the year. Most permafrost has been frozen since thousands of years already. The frozen ground contains half of all the carbon stored in Earth’s soils, despite only covering 15 % of the global soil surface. The top three meters of permafrost soils currently contain already as much carbon as the atmosphere. This huge carbon reservoir is mainly made of dead plant matter that could not be converted into greenhouse gases (yet). Average permafrost temperatures range between -2 and -10 °C in the Arctic and down to -27 °C in Antarctica. Just as we store food in freezers to keep it from spoiling, processes in frozen soil are also slowed down a lot.
Most extremophiles – that’s how we call organisms able to survive in extreme conditions – are microscopic organisms, often consisting of only one cell (unicellular organisms). When water freezes, its salts get separated from the developing water crystals, and form pockets of super salty water (called brine) inside the surrounding frozen ground. Most microbial permafrost life, no matter how extreme, needs these little reservoirs of still liquid water to survive. In addition to the cold, organisms trapped in permafrost have to deal with darkness, geological radiation, low nutrient and energy levels, and high competition with each other for the scarce still digestible organic matter.
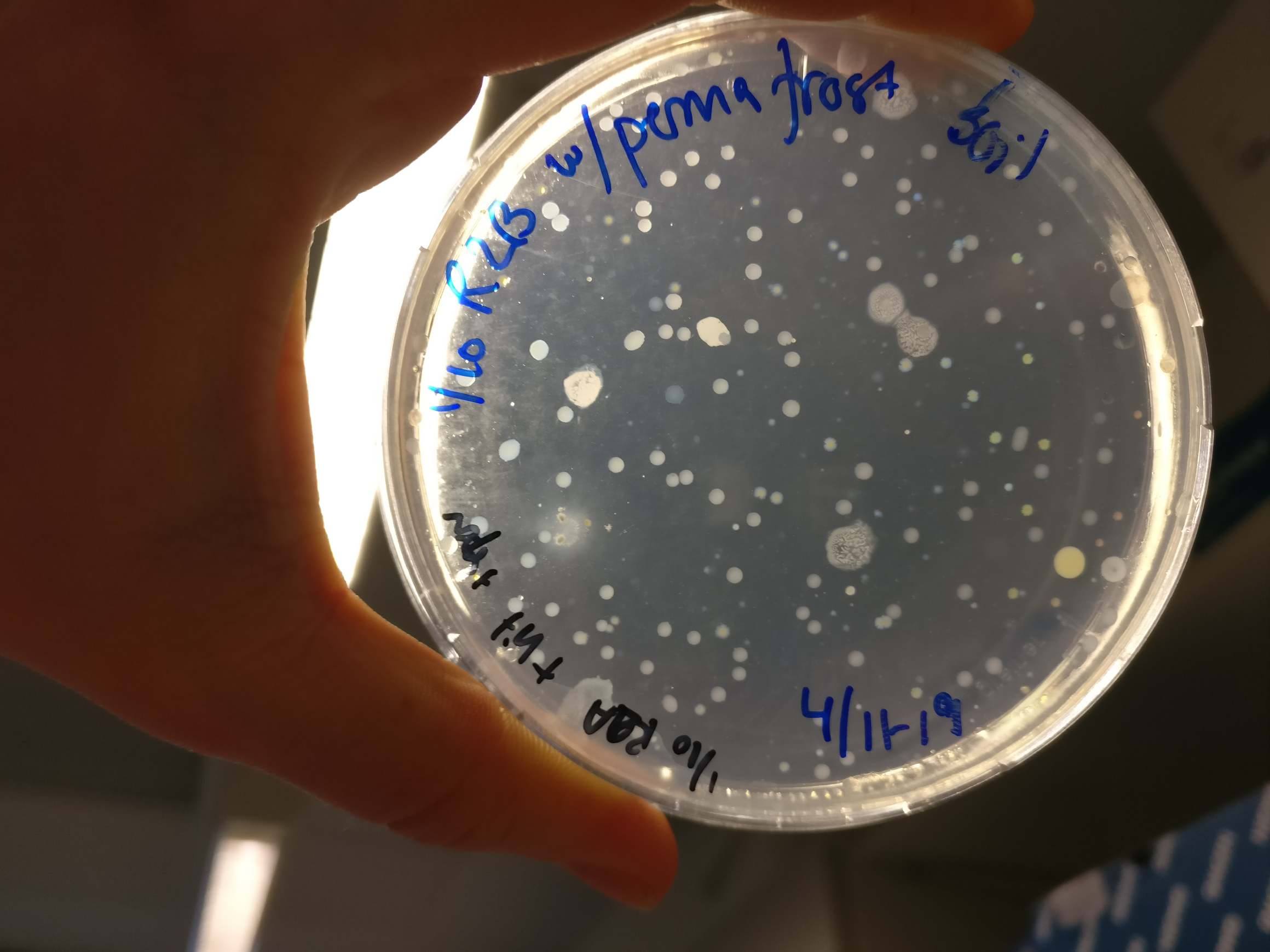
Figure 2: All the milky spots are single colonies of microorganisms grown on a medium in the laboratory. The basis was 26’000-year-old permafrost soil. Maybe the yellow and white spots are two never before documented species, who knows? [credit: Maria Scheel]
Seeing the tiny, ancient survivors: a genetics 101
The first permafrost organisms were found 110 years ago in the Siberian Arctic. But the first species were only characterized by the end of the last century by “cultivating” them at different temperatures and with various food supplies in laboratories. Permafrost soil was infused with a liquid optimum nutrition medium to grow its microorganisms (see Figure 2), but most its microorganisms did not really grow, and a majority stayed unidentified for a long time. It was only with recent genetic insights that scientists were finally able to better identify very ancient species more precisely – without having to grow them first.
Genetics, what? Figure 3 explains, how genes work. Depending on the method used, we can zoom in on different parts of genetics – the whole pool of genes (the DNA), or maybe just those used by a living organism in response to stress it has to deal with (the RNA). As an example: if an organism gets stressed by heat, it can copy the genes of heat shock proteins and translate them into the final protein, which helps our cold microbe to protect the DNA from eroding at higher temperatures. For these approaches, genetic material is extracted from the soils. By sequencing it, the biological information of it (encrypted with the order of four different building blocks, the genetic code) gets converted into bioinformatic signals. Scientists in this field ultimately want to understand with as little bias as possible “Who is there and what are they really doing?”
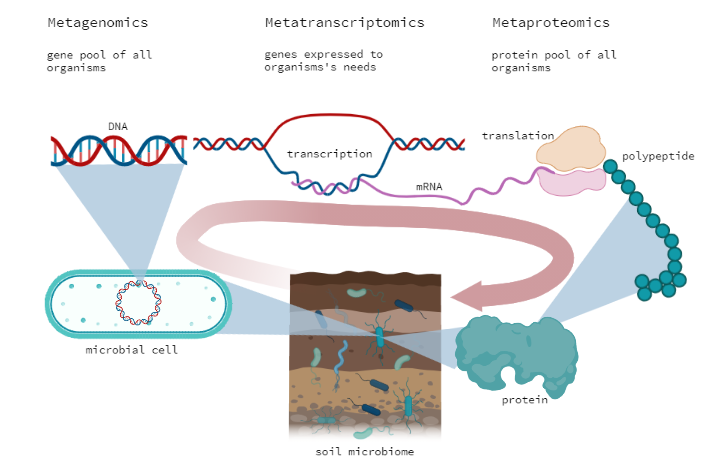
Figure 3: All genes of an organism are pooled in its genome – the DNA. Some of these genes get copied into mRNA (messenger RNA), depending on which gene helps the organism best to respond to its needs. This mRNA is translated into chains of amino acids, which will be folded into the organism’s proteins. Genetic methods are named after which aspect they zoom in on, such as genomics, transcriptomics and proteomics, while “meta” stands for doing this for a whole community, not only one individual. [Credit: Maria Scheel, created via biorender.com]
Now we know how to look – so whom will we find in permafrost?
These methods can be pricy and produce huge datasets but help scientists a lot to find out more about biodiversity in permafrost soils. If you look at the title Figure 1, you can see microbes in intact and eroding permafrost. Some of them are known to survive in permafrost pretty well, such as Bacilli (red) and Firmicutes, that form spores (green). They also quickly grow their population as permafrost thaws, converting freshly available carbon into the greenhouse gases carbon dioxide (CO2) and methane (CH4). Although some other microorganisms can feed on these gases, most might be released to the atmosphere.
There are also the less lucky, such as many Actinobacteria (blue), which are so well adapted to life in the unlikely permafrost habitat, that they might not survive thawing too well. Permafrost also harbours extremophilic archaea, which are another unicellular category of organisms besides bacteria. They seem to enjoy living dangerously all over the globe (e.g., in volcanoes, in acids and in our guts), as well as bacteria, and several multi-cellular organisms, such as fungi (think of the molds on rotten food), but little is known about the latter. The input of fresh and tasty substrates from vegetation decreases with depth and with it the number of organisms that can live with so little. At depth, sometimes the only way to survive is to digest each other’s metabolites (by eating each other’s “poop”!).
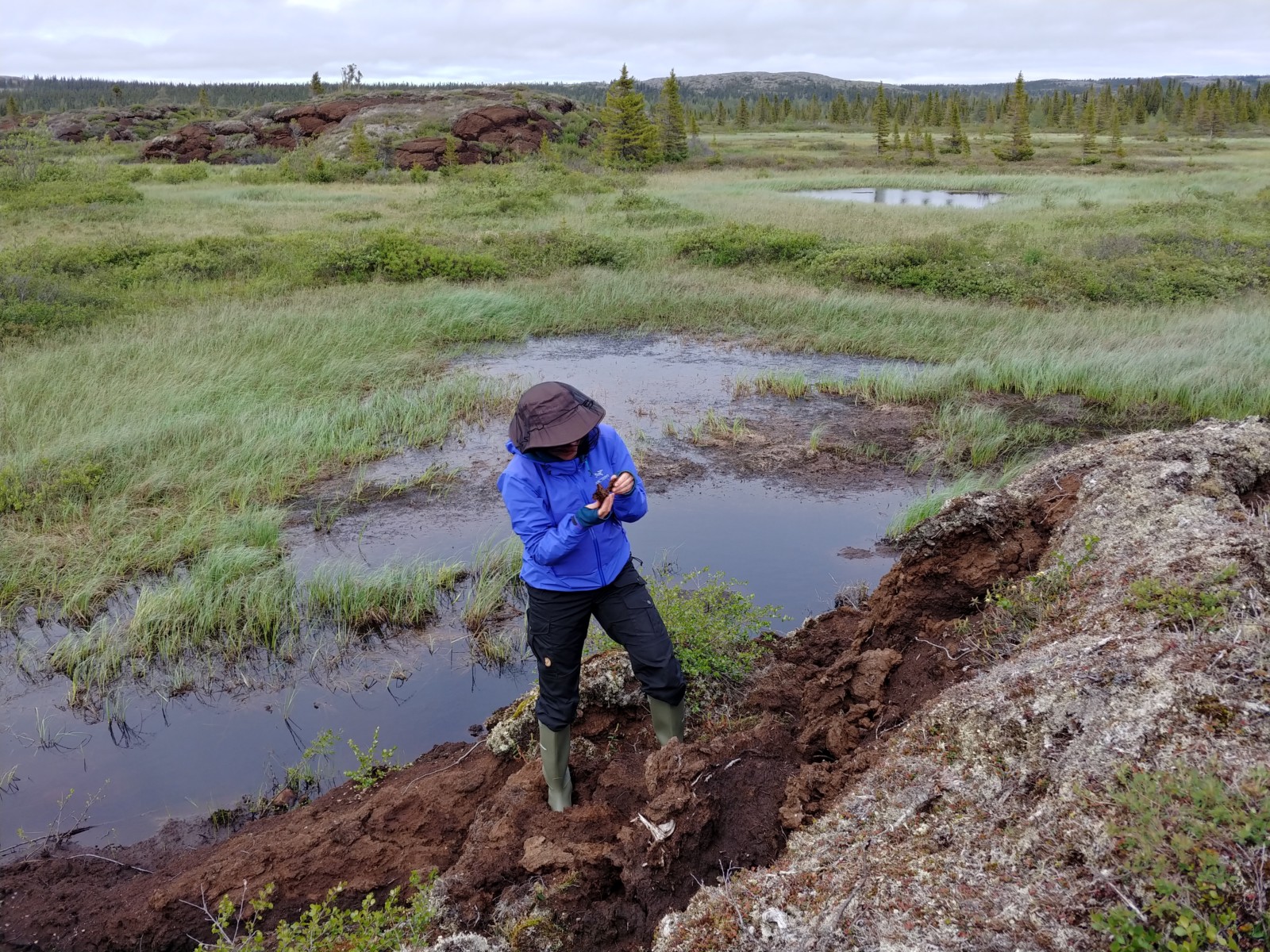
Figure 4: Permafrost soils are quickly thawing and eroding. But what is happening to the invisible microbial life, which remained frozen in it for millennia, when it is getting warmer? [Credit: Pascal Paradis]
How to deal with the permanent stress of life
For a long time, 600’000 years had been accepted to be the age of the oldest DNA derived from living cells. This year though, Katie Sipes and colleagues identified eight genomes from 1 million-year-old (!) permafrost. How can anything survive so long? Among some genes, they found adaptations to long-term energy starvation. The microbes turn down the metabolism to a bare minimum. Imagine you have one bread left until the end of the year – you would eat little and slowly.
In order to survive, permafrost microbes also need to use cold-protection proteins (think of the anti-freeze additive for car motors) as well as very fatty and hence not freezing cell membranes. But permafrost conditions can endanger the cell and its genetic material. The little energy the cell has is put into fixing potential damage from these conditions. Therefore, not enough energy is left to actively multiply and grow the microbial population. Some microbes won’t even try, but just “hibernate”, by forming spores that can survive extreme conditions and switch to full activity again under “better” conditions (who knows it, feels it).
What do these frosty microbes do when “it’s getting hot in here”?
Already a magnificent, alien world, this whole fragile system of surviving crazy microbes is currently changing, as our world is changing. Climate change impacts permafrost through higher temperatures, frequent rain on snow events, as well as an increasing number of wildfires. If this sounds apocalyptic to you, imagine having lived stably for 1 million years! The increasing permafrost temperatures lead to deeper thawing of formerly frozen ground. On top, ground ice, which can be several meters thick ice lenses (as you can see in Figure 5), can melt abruptly (you can read more about permafrost thaw in this previous post).
When it gets too warm, ice lenses melt, supplying a lot of liquid water, but also collapsing the whole soil surface, as you see in Figure 5. This way, ancient carbon reservoirs are freed up: The then water-logged soils favour microbes that can digest organic matter into CO2 (by fermentation) or release CH4 in significant amounts (see Figure 1). When the water has drained out the now collapsed profile, even more CO2 gets released, especially by microbial activity. Currently, up to 15 % of permafrost organic matter stocks are in reach for microbial decomposition, but this number could increase by up to 50 % under an increase of the global temperature by 2 °C.
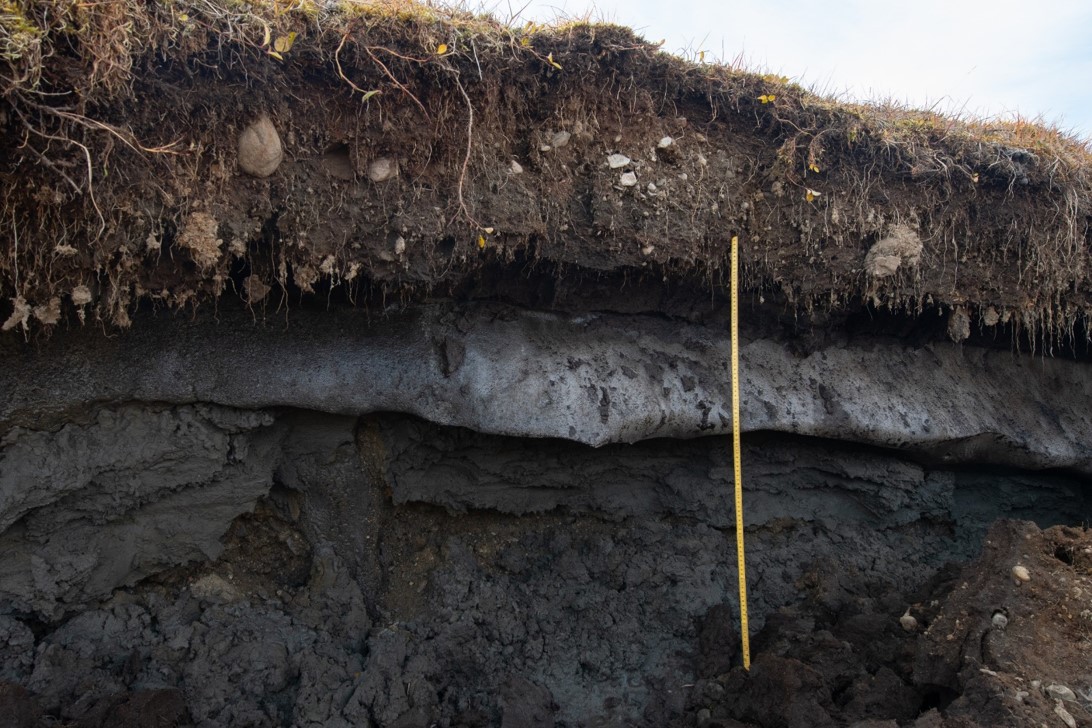
Figure 5: This ice lens in Northeast Greenland formed a long time ago in the ground and was a natural limit to the underlying frozen ground. The grey material is almost 30’000 years old and now not protected from decomposition anymore. [Credit: Torben R. Christensen]
Further reading
- Find our previous blog post about permafrost thaw here
- Cavicchioli et al. (2019) Scientists’ warning to humanity: microorganisms and climate change. Nat. Rev. Microbiol. 17:569–586, DOI:10.1038/s41579-019-0222-5.
- Margesin & Collins (2019) Microbial ecology of the cryosphere (glacial and permafrost habitats): current knowledge. Appl. Microbiol. Biotechnol. 103:2537–2549, DOI:10.1007/s00253-019-09631-3.
- Sipes et al. (2021) Eight Metagenome-Assembled Genomes Provide Evidence for Permafrost. Appl. Environ. Microbiol. 87: e00972-21, DOI:10.1128/AEM.00972-21.
Edited by Clara Burgard and Giovanni Baccolo
Maria Scheel is a PhD student at Aarhus University in Denmark, where she works tightly linked with the Greenland Ecosystem Monitoring and the Arctic Research Centre. Despite a Marine Biologist by education, her passion for genetics brought her to the terrestrial realm, where her current research explores how permafrost microorganisms adapt to the ongoing thaw and erosion in Northeast Greenland Besides that, she currently is a representative for APECS Denmark and recently started her blog Mindful Scientist, sharing experiences and raising awareness about a healthy mind at work. She tweets as @Maria_Scheel_. Contact Email: maria.scheel@ecos.au.dk.