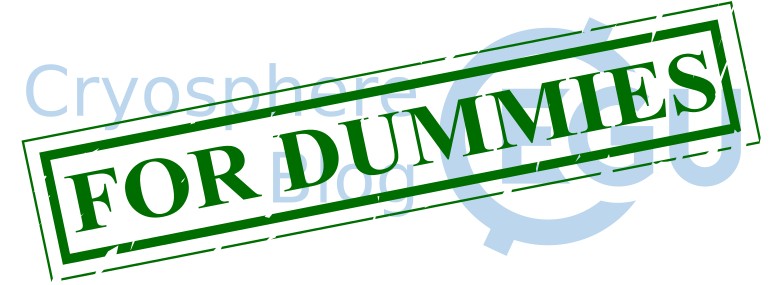
MISI is a term that is often thrown into dicussions and papers which talk about the contribution of Antarctica to sea-level rise but what does it actually mean and why do we care about it?
MISI stands for Marine Ice Sheet Instability. In this article, we are going to attempt to explain this term to you and also show you why it is so important.
Background
The Antarctic Ice Sheet represents the largest potential source of future sea-level rise: if all its ice melted, sea level would rise by about 60 m (Vaughan et al., 2013). According to satellite observations, the Antarctic Ice Sheet has lost 1350 Gt (gigatonnes) of ice between 1992 and 2011 (1 Gt = 1000 million tonnes), equivalent to an increase in sea level of 3.75 mm or 0.00375 m (Shepherd et al., 2012). 3.75 mm does not seem a lot but imagine that this sea-level rise is evenly spread over all the oceans on Earth, i.e. over a surface of about 360 million km², leading to a total volume of about 1350 km³, i.e. 1350 Gt of water… The loss over this period is mainly due to increased ice discharge into the ocean in two rapidly changing regions: West Antarctica and the Antarctic Peninsula (Figure 1, blue and orange curves respectively).
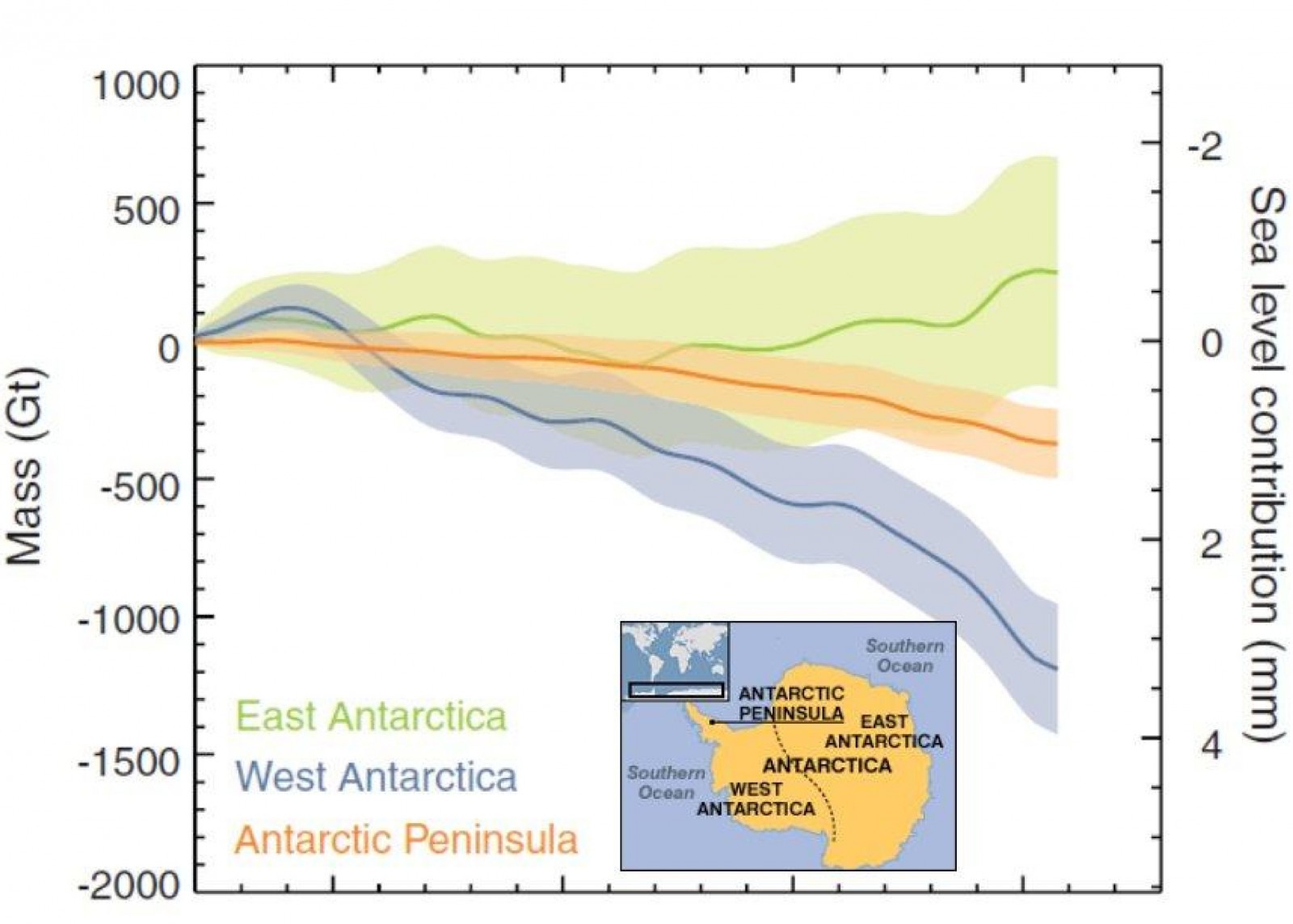
Figure 1: Cumulative ice mass changes (left axis) and equivalent sea-level contribution (right axis) of the different Antarctic regions based on different satellite observations (ERS-1/2, Envisat, ICESat, GRACE) from 1992 to 2011 (source: adapted from Fig. 5 of Shepherd et al., 2012 ) with addition of inset: Antarctic map showing the different regions ( source )
What are the projections for the future?
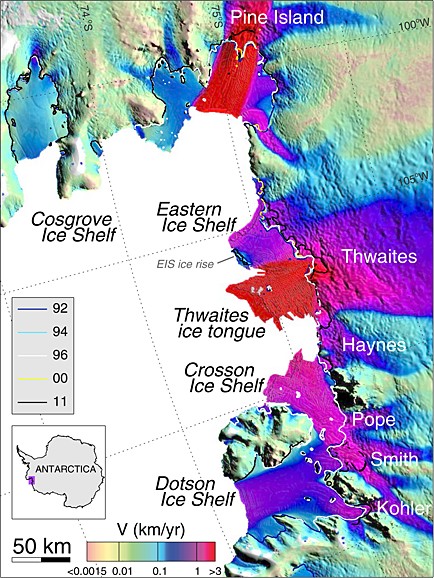
Figure 2: Ice velocity of the glaciers in the Amundsen Sea Embayment, West Antarctica, using ERS-1/2 radar data in winter 1996. The grounding line (boundary between ice sheet and ice shelf) is shown for 1992, 1994, 1996, 2000 and 2011 (source: Fig. 1 of Rignot et al., 2014 ).
According to model projections from the Intergovernmental Panel on Climate Change (IPCC), global mean sea level will rise by 0.26 to 0.82 m during the twenty-first century (Church et al., 2013). The contribution from the Antarctic Ice Sheet in those projections will be about 0.05 m (or 50 mm) sea-level equivalent, i.e. 10% of the global projected sea-level rise, with other contributions coming from thermal expansion (40 %), glaciers (25 %), Greenland Ice Sheet (17 %) and land water storage (8 %).
The contribution from Antarctica compared to other contributions does not seem huge, however there is a high uncertainty coming from the possible instability of the West Antarctic Ice Sheet. According to theoretical (Weertman, 1974; Schoof, 2007) and recent modeling results (e.g. Favier et al., 2014; Joughin et al., 2014), this region could be subject to marine ice sheet instability (MISI), which could lead to considerable and rapid ice discharge from Antarctica. Satellite observations show that MISI may be under way in the Amundsen Sea Embayment (Rignot et al., 2014), where some of the fastest flowing glaciers on Earth are located, e.g. Pine Island and Thwaites glaciers (Figure 2). So what exactly is MISI?
What is marine ice sheet instability (MISI)?
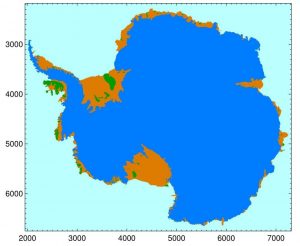
Figure 3: Antarctic map of ice sheet (blue), ice shelves (orange) and islands/ice rises (green) based on satellite data (ICESat and MODIS). The grounding line is the separation between the ice sheet and the ice shelves. Units on X and Y axes are km (source: NASA ).
To understand the concept of MISI, it is important to define both ‘marine ice sheet’ and ‘grounding line’:
- A marine ice sheet is an ice sheet sitting on a bedrock that is below sea level, for example the West Antarctic Ice Sheet.
- The grounding line is the boundary between the ice sheet, sitting on land, and the floating ice shelves (Figure 3 for a view from above and Figure 4 for a side view). The position and migration of this grounding line control the stability of a marine ice sheet.
The MISI hypothesis states that when the bedrock slopes down from the coast towards the interior of the marine ice sheet, which is the case in large parts of West Antarctica, the grounding line is not stable (in the absence of back forces provided by ice shelves, see next section for more details). To explain this concept, let us take the schematic example shown in Figure 4:
- The grounding line is initially located on a bedrock sill (Figure 4a). This position is stable: the ice flux at the grounding line, which is the amount of ice passing through the grounding line per unit time, matches the total upstream accumulation.
- A perturbation is applied at the grounding line, e.g. through the incursion of warm Circumpolar Deep Water (CDW, red arrow in Figure 4) below the ice shelf as observed in the Amundsen Sea Embayment.
- These warm waters lead to basal melting at the grounding line, ice-shelf thinning and glacier acceleration, resulting in an inland retreat of the grounding line.
- The grounding line is then located on a bedrock that slopes downward inland (Figure 4b), i.e. an unstable position where the ice column at the grounding line is thicker than previously (Figure 4a). The theory shows that ice flux at the grounding line is strongly dependent on ice thickness there (Weertman, 1974; Schoof, 2007), so a thicker ice leads to a higher ice flux.
- Then, the grounding line is forced to retreat since the ice flux at the grounding line is higher than the upstream accumulation.
- This is a positive feedback and the retreat only stops once a new stable position is reached (e.g. a bedrock high), where both ice flux at the grounding line and upstream accumulation match.
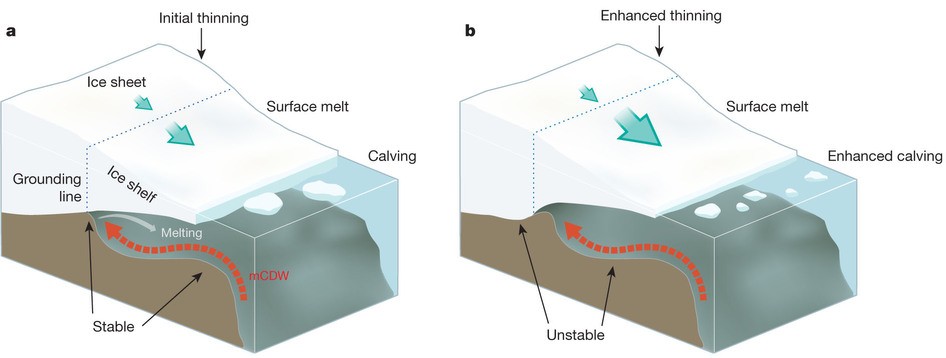
Figure 4: Schematic representation of the marine ice sheet instability (MISI) with (a) an initial stable grounding-line position and (b) an unstable grounding-line position after the incursion of warm Circumpolar Deep Water (CDW) below the ice shelf (source: Fig. 3 of Hanna et al., 2013 ).
- In summary, the MISI hypothesis describes the condition where a marine ice sheet is unstable due to being grounded below sea level on land that is sloping downward from the coast to the interior of the ice sheet.
- This configuration leads to potential rapid retreat of the grounding line and speed up of ice flow from the interior of the continent into the oceans.
Is there evidence that MISI is happening right now?
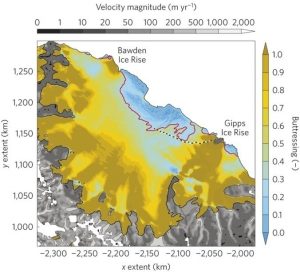
Figure 5: Buttressing provided by Larsen C ice shelf, Antarctic Peninsula, based on a model simulation (Elmer/Ice). Buttressing values range between 0 (no buttressing) and 1 (high buttressing). The red contour shows the buttressing=0.3 isoline. Observed ice velocity is also shown (source: Fig. 2 of Fürst et al., 2016 ).
In reality, the grounding line is often stabilized by an ice shelf that is laterally confined by side walls (see Figure 5, where Bawden and Gipps ice rises confine Larsen C ice shelf) or by an ice shelf that has a contact with a locally grounded feature (Figure 6). Both cases transmit a back force towards the ice sheet, the ‘buttressing effect’, which stabilizes the grounding line (Goldberg et al., 2009; Gudmundsson, 2013) even if the configuration is unstable, i.e. in the case of a grounding line located on a bedrock sloping down towards the interior (Figure 4b).
However, in the last two decades, the grounding lines of the glaciers in the Amundsen Sea Embayment (Pine Island and Thwaites glaciers for example) retreated with rates of 1 to 2 km per year, in regions of bedrock sloping down towards the ice sheet interior (Rignot et al., 2014). The trigger of these grounding-line retreats is the incursion of warm CDW penetrating deeply into cavities below the ice shelves (Jacobs et al., 2011), carrying important amounts of heat that melt the base of ice shelves (Figure 4). Increased basal melt rates have led to ice-shelf thinning, which has reduced the ice-shelf buttressing effect and increased ice discharge. All of this has led to grounding-line retreat. The exact cause of CDW changes is not clearly known but these incursions are probably linked to changes in local wind stress (Steig et al., 2012) rather than an actual warming of CDW.
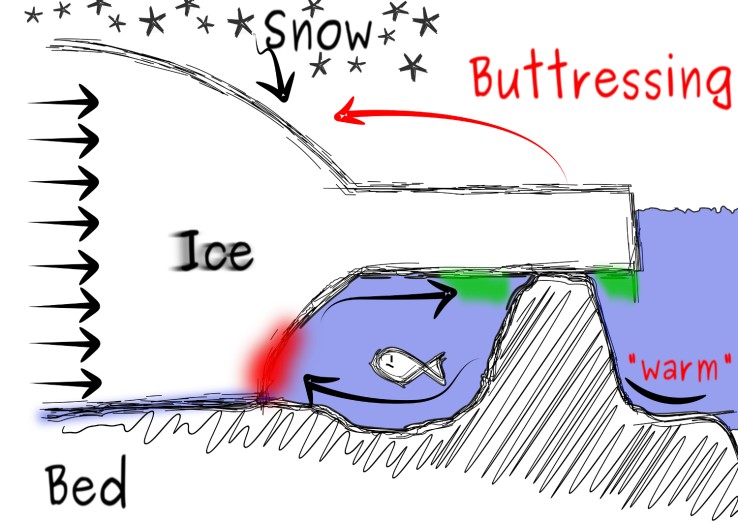
Figure 6: Schematic representation of ice-shelf buttressing by a local pinning point (source: courtesy of R. Drews ).
There is currently no major obstacle to these grounding line retreats. Therefore, the Amundsen Sea Embayment is probably experiencing MISI and glaciers will continue to retreat if no stabilization is reached. This sector of West Antarctica contains enough ice to raise global sea level by 1.2 m.
What can we do about it?
MISI is probably ongoing in some parts of Antarctica and sea level could rise more than previously estimated if the grounding lines of the glaciers in the Amundsen Sea Embayment continue to retreat so fast. This could have catastrophic impacts on populations living close to the coasts, for example more frequent flooding of coastal cities, enhanced coastal erosion or changes in water quality.
Thus, it is important to continue monitoring the changes happening in Antarctica, and particularly in West Antarctica. This will allow us to better understand and project future sea-level rise from this region, as well as better adapt the cities of tomorrow.
Edited by Clara Burgard and Emma Smith
David Docquier is a post-doctoral researcher at the Earth and Life Institute of Université catholique de Louvain (UCL) in Belgium. He works on the development of processed-based sea-ice metrics in order to improve the evaluation of global climate models (GCMs). His study is embedded within the EU Horizon 2020 PRIMAVERA project, which aims at developing a new generation of high-resolution GCMs to better represent the climate.
William Hughes-Games
Calling it an incursion of the slightly warmer circumpolar sea water may be missing the dynamics of the system. As this warmer water melts the ice front, the water in contact with the ice becomes fresher and hence lighter than the surrounding water and hence buoyant. Clearly it would rise up along the sloping ceiling of ice and flow out on the surface of the ocean beyond the edge of the ice. Some of this fresher water would cool and freeze on to the bottom of the ice shelf which might help explain the increase in Marine ice in the Antarctic in recent years. Of more importance as this water flows out on the surface of the ocean it would cause more water to be sucked in from the depths of the surrounding ocean. It is almost an Air Lift effect except this is a water lift and is only confined on one side unlike a classical air lift. As the grounding line gets deeper and deeper, one would expect the effect to increase and it will also be aided by the dense salty water flowing down the retrograde slope. With such a system we could see a more rapid melting of the ice than suggested by all the models.
Pingback: Cryospheric Sciences | Water Masses “For Dummies”
Pingback: Cryospheric Sciences | Image of The Week – The Pulsating Ice Sheet!
Pingback: A(68)nniversary – Atmosphere and climate over Larsen C
Pingback: Cryospheric Sciences | Image of the Week – Oh Sheet!
Pingback: Cryospheric Sciences | Ice-hot news: The cryosphere and the 1.5°C target
Pingback: Ninovus | A Terrifying Sea-Level Prediction Now Looks Far Less Likely
Pingback: Cryospheric Sciences | Image of the Week – Delaying the flood with glacial geoengineering
Pingback: Cryospheric Sciences | Image of the Week — Cavity leads to complexity
Pingback: Cryospheric Sciences | Image of the Week – Kicking the ice’s butt(ressing)
Pingback: Cryospheric Sciences | Ice-hot news: The IPCC Special Report on the Oceans and the Cryosphere under Climate Change
Pingback: Cryospheric Sciences | Water plumes are tickling the Greenland Ice Sheet
Pingback: Cryospheric Sciences | What’s up on Thwaites Glacier?
Pingback: What’s up on Thwaites Glacier? - Scientists4Climate
Pingback: Cryospheric Sciences | How do the ups and downs of the solid Earth influence the future of the West Antarctic ice sheet?
Pingback: Cryospheric Sciences | Hysteresis For Dummies – Why history matters
Pingback: Cryospheric Sciences | How small changes can make a big difference: tipping points in Antarctica
Pingback: Cryospheric Sciences | The “Cliffs Notes” on Ice-Cliff Failure
Pingback: The world’s biggest ice sheet is more vulnerable to global warming than scientists previously thought – News Worlds
Pingback: The world's greatest ice sheet is extra weak to international warming than scientists beforehand thought | Hull News Today
Pingback: The world's biggest ice sheet is more vulnerable to global warming than scientists previously thought | Reading News Today
Pingback: The world's biggest ice sheet is more vulnerable to global warming than scientists previously thought | Manchester News Today
Pingback: The world's biggest ice sheet is more vulnerable to global warming than scientists previously thought
Pingback: The world's biggest ice sheet is more vulnerable to global warming than scientists previously thought | Nottingham News Today
Pingback: The world’s biggest ice sheet is more vulnerable to global warming than scientists previously thought – The Conversation – Menopausal Mother Nature
Pingback: The world's largest ice sheet is extra susceptible to international warming than scientists beforehand thought | Stoke News Today
Pingback: The world's greatest ice sheet is extra susceptible to international warming than scientists beforehand thought| Sunderland News Today
Pingback: The world's largest ice sheet is extra weak to world warming than scientists beforehand thought | Glasgow News Today
Pingback: The world's biggest ice sheet is more vulnerable to global warming than scientists previously thought | Birmingham News Today
Pingback: The world's biggest ice sheet is more vulnerable to global warming than scientists previously thought | Bradford News Today
Pingback: The world's biggest ice sheet is more vulnerable to global warming than scientists previously thought | Derby News Today
Pingback: The world's biggest ice sheet is more vulnerable to global warming than scientists previously thought - Newspostalk - Global News Platfrom
Pingback: The world’s largest ice cap is more vulnerable to global warming than scientists previously thought – akhbar4you
Pingback: The world’s biggest ice sheet is more vulnerable to global warming than scientists previously thought
Pingback: The world’s largest ice sheet is more vulnerable to global warming than scientists previously thought – koura4u
Pingback: The world's biggest ice sheet is more vulnerable to global warming than scientists previously thought - HuntingHeadlines
Pingback: “Un gigante dormido”: la capa de hielo más grande del mundo podría colapsar e inundar gran parte del planeta – My Blog
Pingback: The world’s biggest ice sheet is more vulnerable to global warming than scientists previously thought - Philippine Canadian Inquirer Nationwide Filipino Newspaper
Pingback: “Un gigante dormido”: la capa de hielo más grande del mundo podría colapsar e inundar gran parte del planeta – Refresh.news
Pingback: The world's biggest ice sheet is more vulnerable to global warming than scientists previously thought - Samachar Central
Pingback: The world's biggest ice sheet is more vulnerable to global warming than scientists previously thought - TheDailyCheck.net
Pingback: The world's biggest ice sheet is more vulnerable to global warming than scientists previously thought - TechCodex
Pingback: The world's biggest ice sheet is more vulnerable to global warming than scientists previously thought - My Droll
Pingback: The world's biggest ice sheet is more vulnerable to global warming than scientists previously thought » NewsTale
Pingback: The world's largest ice sheet is more vulnerable to global warming than scientists thought - zero coverage - Zero Coverage
Pingback: The world's biggest ice sheet is more vulnerable to global warming than scientists previously thought - newscontinue
Pingback: The world’s largest ice sheet is more vulnerable to global warming than scientists previously thought – Mc Cafe Retail News
Pingback: The world's biggest ice sheet is more vulnerable to global warming than scientists previously thought - Verve times
Pingback: The world's biggest ice sheet is more vulnerable to global warming than scientists previously thought - SecularTimes
Pingback: The world's biggest ice sheet is more vulnerable to global warming than scientists previously thought - swifttelecast
Pingback: The world's biggest ice sheet is more vulnerable to global warming than scientists previously thought - TodaysChronic.com
Pingback: The world's largest ice sheet is more vulnerable to global warming than scientists thought - trending town
Pingback: The world's largest ice sheet is more vulnerable to global warming than scientists thought - think news - think news
Pingback: The world’s biggest ice sheet isworse than scientists previously thought - Brighton Journal
Pingback: THE CONVERSATION: Global warming putting world’s biggest ice sheet at greater risk than scientists previously thought - Country Highlights
Pingback: DIE GESPREK: Aardverwarming plaas die wêreld se grootste ysplaat groter gevaar as wat wetenskaplikes voorheen gedink het - YouAreMy
Pingback: THE CONVERSATION: Global warming putting world’s biggest ice sheet at greater risk than scientists previously thought - powermeentrepreneurs
Pingback: Global warming threatening world’s biggest ice sheet… – Daily Maverick – Menopausal Mother Nature
Pingback: War THE CONVERSATION: Global warming putting world’s biggest ice sheet at greater risk than scientists previously thought – U-News
Pingback: THE CONVERSATION: Global warming putting world’s biggest ice sheet at greater risk than scientists previously thought – A23 Tips
Pingback: THE CONVERSATION: Global warming putting world’s biggest ice sheet at greater risk than scientists previously thought read more at worldnews365.me - World News 365
Pingback: The world’s biggest ice sheet is more vulnerable to global warming than scientists previously thought – EastMojo – ONE News
Pingback: La capa de hielo más grande del mundo es más vulnerable al calentamiento global de lo que pensaban los científicos - Notiulti
Pingback: The world’s biggest ice sheet is more vulnerable to global warming than scientists previously thought - zoeconsidine
Pingback: The world’s biggest ice sheet is more vulnerable to global warming than scientists previously thought - OGC News
Pingback: Antarctica: The world’s biggest ice sheet is more vulnerable to global warming than scientists previously thought - southatlanticnews