The retreat of large glaciers that drain the Greenland and Antarctic ice sheets could expose immense ice-cliffs at newly-bared calving faces, which are the exposed ends of glaciers where, in these cases, glacier ice meets the ocean. Past a certain height, these ice cliffs will become susceptible to collapsing from high stresses, a process known as structural ice-cliff failure. If a taller ice clif ...[Read More]
The “Cliffs Notes” on Ice-Cliff Failure
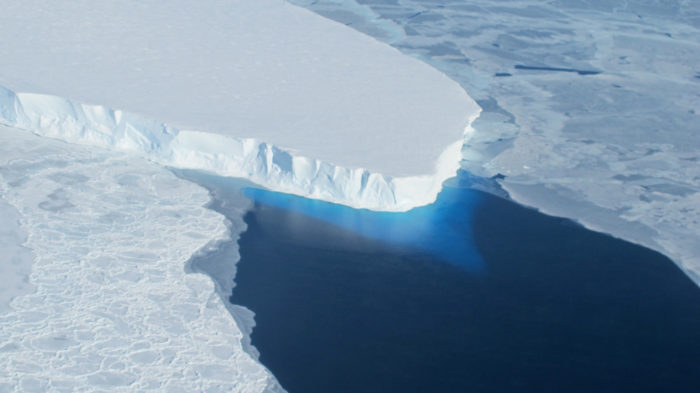