Ice caps, valley glaciers, cirque glaciers, piedmont glaciers, ice sheets… I’m guessing that if you are a glaciology enthusiast, you have already heard about these types of glaciers. But you probably don’t know anything about regenerated glaciers, am I right? Well, you are in the right place! Let’s find out more about this little-known glacier type. Classifying glaciers Glaciers are classified on ...[Read More]
Did you know…about regenerated glaciers?
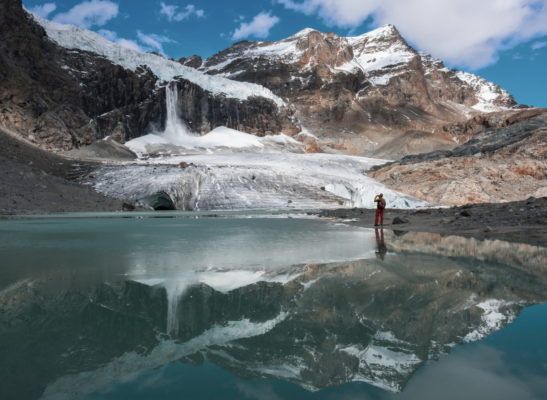