Plumbing is something we take for granted: the pipes that bring us water to wash and drink, and the pipes that take the waste water away again. We see the taps and basins in our kitchen and bathroom – but the pipes are hidden away under the floor or inside the walls – and we mostly ignore them until there’s a leak or a blockage!
It turns out that glaciers have plumbing too – and like the pipes in our streets, most of it is hidden beneath our feet. If you walk on a glacier in the summertime, you will see streams (and sometimes rivers) of liquid water running over the ice. This is usually meltwater: snow or surface ice that has been melted by the heat from the sun. If you follow one of these streams, it often disappears into a hole in the ice – like going down the plughole in your sink. These holes are called “moulins” – from the French for “mill”, because they resemble the fast-flowing water in a traditional watermill). So, where does the water go? We don’t really know!
A large moulin on Sermeq Kujalleq (Store Glacier) in Western Greenland. The water plunging into the glacier sends up a spray of droplets, causing the rainbow seen here. [Credit: Mike Prior-Jones, with thanks to the RESPONDER project]
Where does the water go?
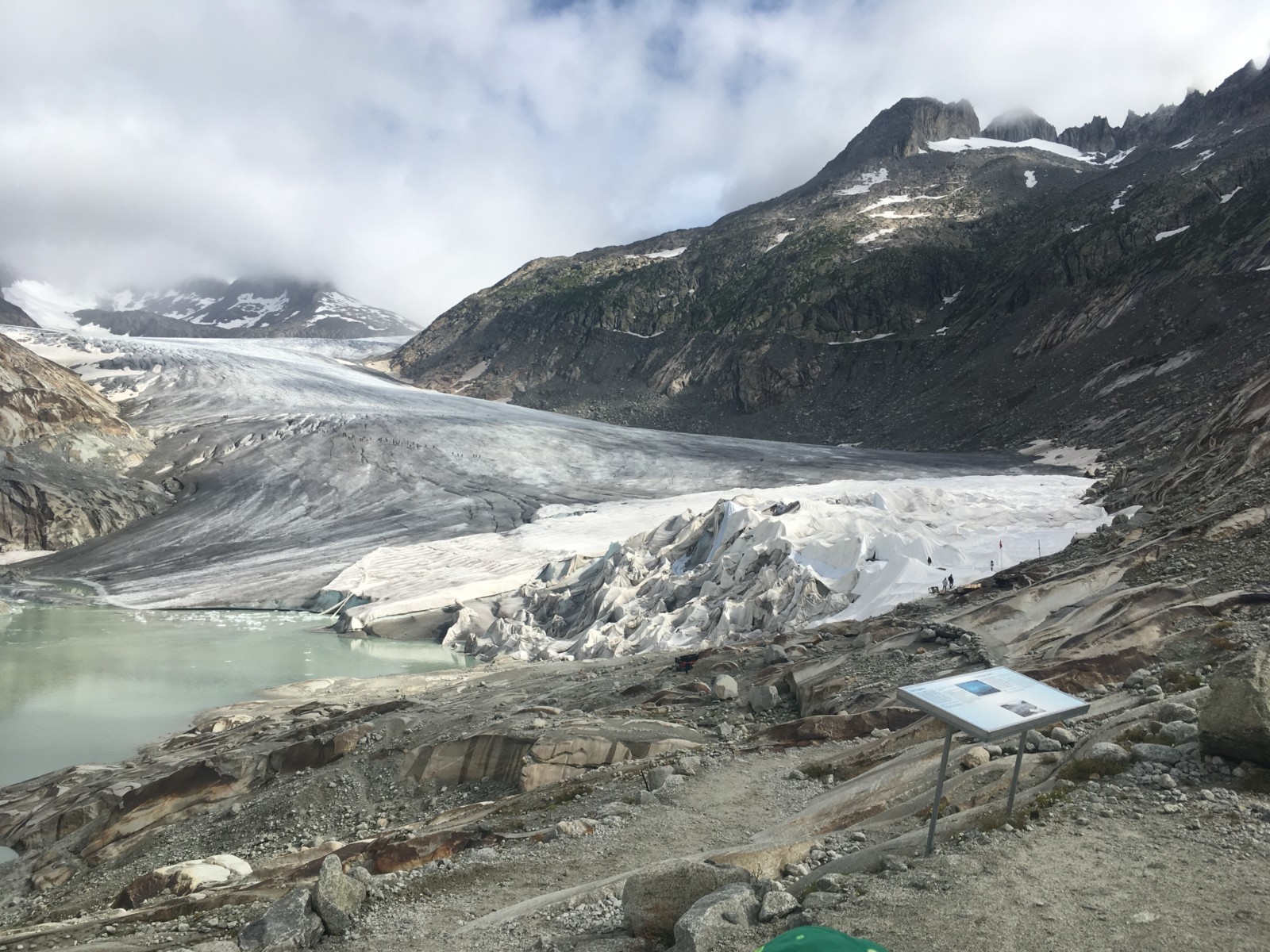
The Rhône Glacier (Switzerland) and its proglacial lake. The white sheeting is fleece fabric placed over the glacier to reduce its melt rate – there is a commercially-run ice cave underneath. [Credit: Mike Prior-Jones]
What we do know is that the water somehow travels through or beneath the glacier and then emerges at the low end of the glacier, which is called the terminus. It’s quite common to find a lake (a “proglacial lake”) at the terminus of glaciers in mountain valleys and most of the water flowing through these lakes is meltwater that emerges from under the glacier. On smaller mountain glaciers we have a clearer picture of how the water flows – we know that it makes its way towards the bed, and depending on the time of year it does two different things. Early in the spring the water forms a thin, high-pressure layer underneath the glacier, which actually lifts the glacier ice up (this is called “hydraulic jacking”). Later in the year, the water cuts and melts bigger channels in the bottom of the glacier ice, and these channels generally then connect to moulins and crevasses, which act as air vents as well as bringing water down into the channels. Because the channels are connected to the air, the water pressure becomes much lower.
What we really want to understand is how this process works in more detail, and especially how it works on large ice sheets such as in Greenland.
Exploring under glaciers
The trouble is, accessing the environment underneath the glacier is very tricky. Moulins are very hazardous – potentially full of fast-flowing water, so exploring them directly by diving is not an option. People do explore old, dry moulins (akin to caving) but this is also pretty hazardous and the opportunities to do it safely are quite limited. So, most studies of this environment instead make use of sensors. Just as space scientists build space probes to explore the solar system and report back to Earth, we can build “subglacial probes” to explore the hidden plumbing of the glaciers.
Subglacial probes have their own challenges. Most commonly scientists drill a borehole – either mechanically, or with a “hot water drill”, which is essentially a giant pressure-washer. Then they lower probes down the borehole on the end of a cable. The cable provides power to the sensors and brings back the data to a computer on the surface. However, this doesn’t always work well: firstly, in fast-flowing glaciers the ice motion breaks the cable after a relatively short period of time (typically a few weeks or months). Cabled sensors also don’t work well in moulins: the swirling, fast-flowing water tends to tie the cable into knots and eventually it breaks.
So, what if we do without the cable? Can we have a wireless subglacial probe?
Introducing Cryoegg
Yes, we can – and I’ve spent the last year-and-a-half designing and building one. This is Cryoegg – the wireless subglacial probe that Liz Bagshaw and I have developed at Cardiff University in Wales, UK.
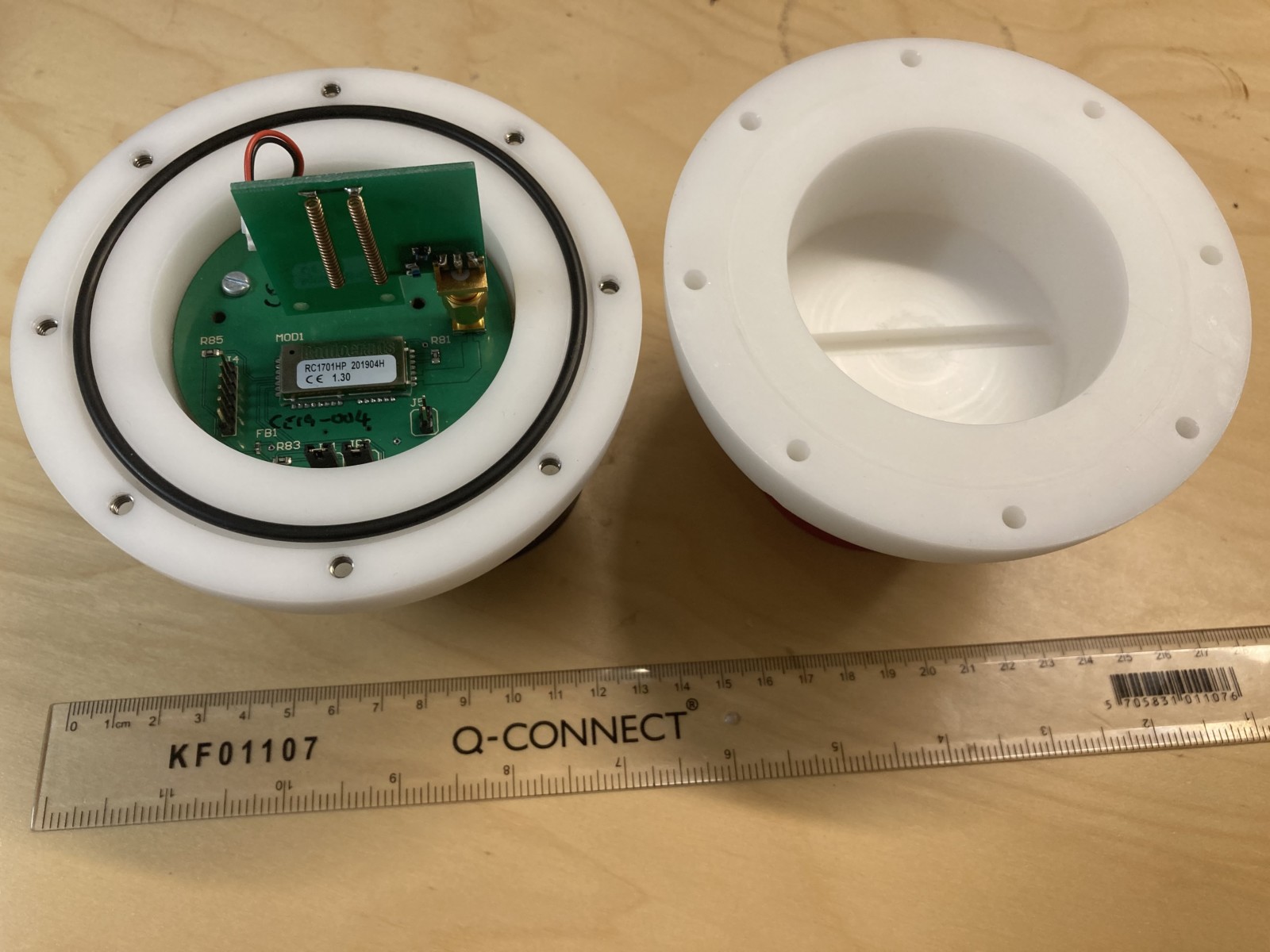
Cryoegg, shown with the upper half of the case removed to reveal the internal electronics. [Credit: Mike Prior-Jones]
Cryoegg is nearly spherical, because we want it to travel easily through the water channels within the glacier and not get caught or snagged on anything. We can deploy it either by dropping it into a moulin, or lowering it down a borehole. It carries its own internal battery, sensors, and a radio transmitter. Glacier ice is actually fairly transparent to radio waves and lots of glaciologists already use radar to look through the ice and see the shape of the glacier bed (e.g. see this previous post). This means that we can also use radio signals to carry the data from our sensors up to the surface. Our receiving antenna picks up the signal coming from Cryoegg and we can see the data from the sensors in real time. We tested it in Greenland last summer (in the borehole drilled for the EastGRIP ice core) and were able to pick up the signal from more than 1.3km below the ice!
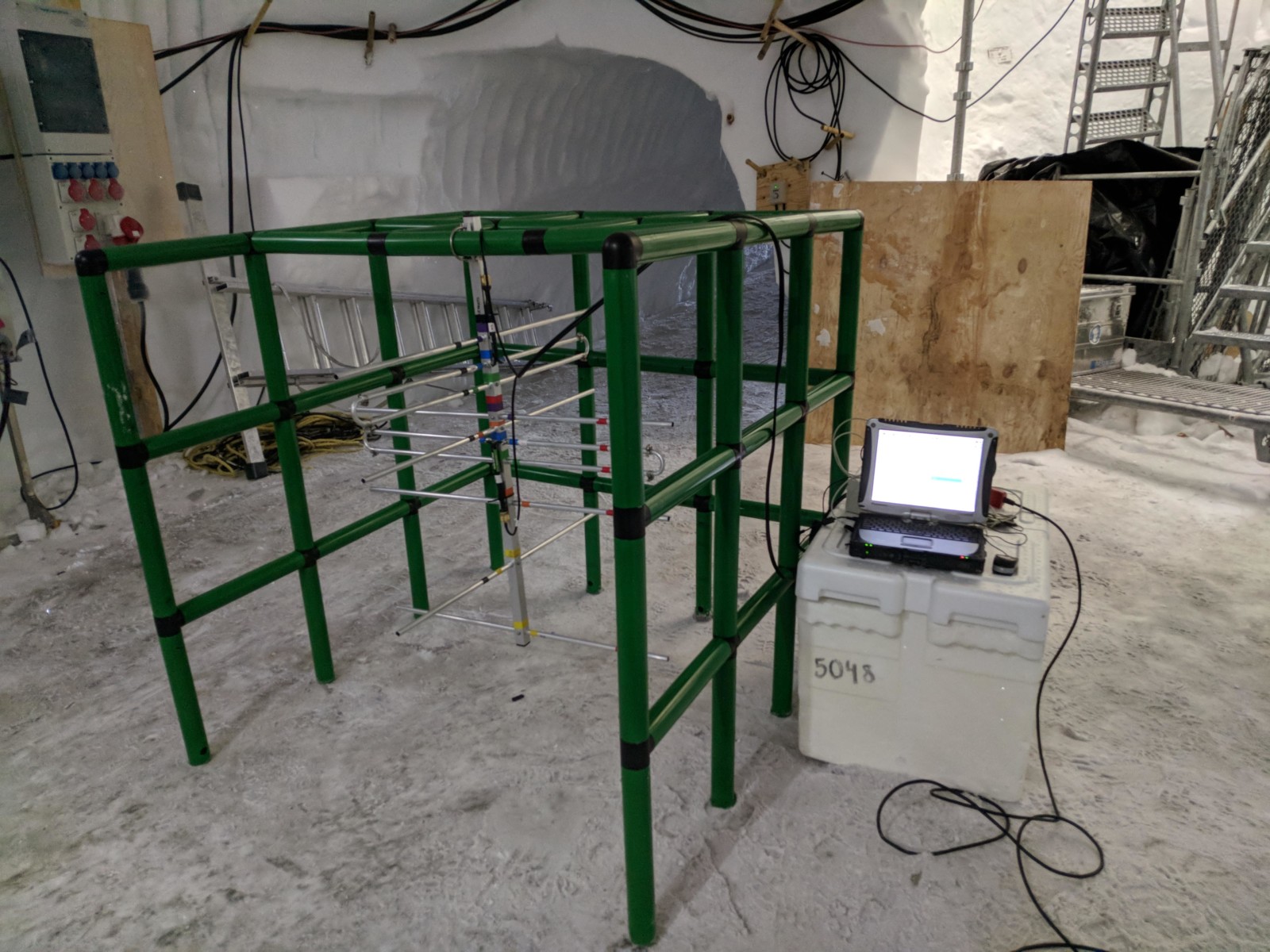
The Cryoegg receiving setup at the EastGRIP drill site. The signal is received by the antenna and decoded in real time on the laptop. [Credit: Liz Bagshaw, with thanks to the EastGRIP project]
Cryoegg has three sensors: a temperature sensor, a pressure sensor, and an electrical conductivity sensor. What’s that last one? Well, glacier meltwater is extremely pure – it’s effectively freeze-distilled – and pure water does not conduct electricity very well. However, as the water comes into contact with other materials (rocks, sand, soil, clay, etc) it dissolves the minerals they contain and this increases its conductivity. So if we see low conductivity, we know the water is freshly melted, but if we see higher conductivity, then we know that Cryoegg has encountered old, slow-moving water that’s had lots of time to pick up minerals from the glacier bed.
A field trial in Switzerland
We had a field trial on the Rhône Glacier in Switzerland in August 2019 where we were using small moulins that had been created artificially. Mauro Werder from ETH Zürich made these moulins by drilling a hole in the glacier with a hot-water drill, and then diverting a stream into the hole, creating a new moulin. We lowered Cryoegg into the moulin on the end of a rope (this was just for testing, so that we could get it back!).
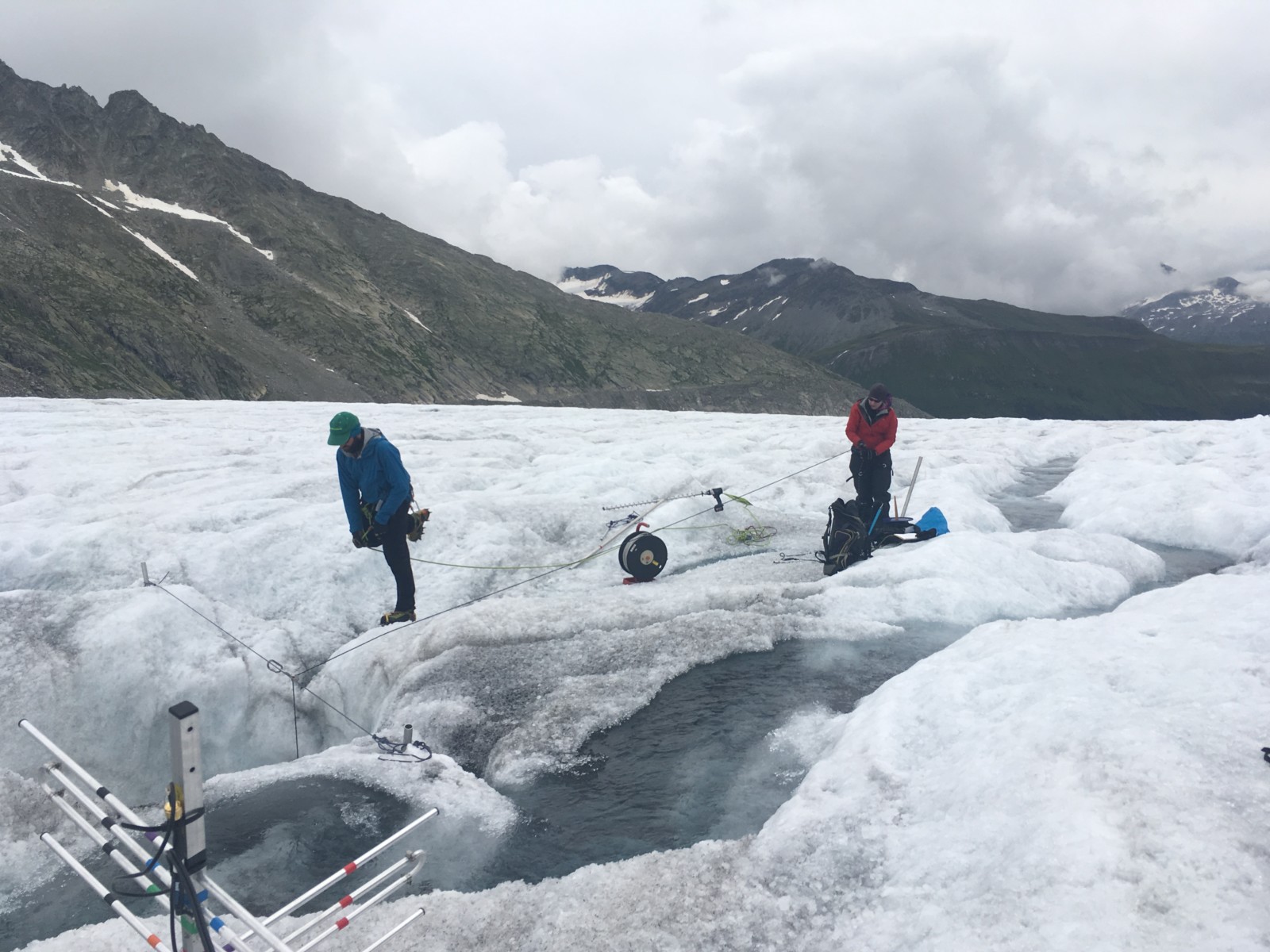
Liz Bagshaw and Mauro Werder lowering Cryoegg into the moulin on the Rhône glacier [Credit: Mike Prior-Jones]
Once Cryoegg was in the bottom of the moulin, we could see the water pressure from the sensor: initially, the sensor indicated that Cryoegg was sitting in more than 25m depth of water. Over about an hour, the pressure reading steadily dropped to zero, indicating that water was draining out of the moulin. All the while, the stream at the top of the moulin was continuing to add water, so something was making the moulin drain away faster. This effect has been observed many times before, going back to work by the Swiss glaciologist Hans Röthlisberger in the 1970s (Röthlisberger and Lang, 1987).
We also tried another experiment: adding salt to the water in the stream feeding the moulin. Ordinary table salt (sodium chloride) dissolves very easily in water and will increase its electrical conductivity (EC). We put a sensor in the stream which could record the EC. Then we added the salt upstream of the sensor. What you see on the sensor is a sudden spike in EC – the “slug” of salty water rushes past the sensor and then the EC returns to normal. What was interesting was that we could also see the EC measurements from Cryoegg. Sure enough, we saw the EC reading from Cryoegg peak about a minute later than the stream sensor. This “lag time” gives us an indication of the speed that the stream and moulin were flowing: 2.75ms-1, which is 10km/h. We can also calculate the “discharge” from the EC measurements – this tells us how much water is flowing per second, and we can see that we’re measuring about a hundred litres per second – slightly more in the bottom of the moulin because it’s fed by a second smaller stream as well as the one we were measuring. Whilst these measurements aren’t particularly interesting in themselves – people have measured moulins before by similar methods – what is exciting is the idea that we can see the data from the bottom of the moulin in real time, and compare it with the surface stream. That gives us a much clearer picture of how the surface conditions connect with the subglacial conditions.
Future plans
In the future, we’re planning to put Cryoeggs underneath glaciers and leave them to report data as they drift through the water channels. That’ll give us a really new insight into their “hidden plumbing”. We’re planning to enhance the design to increase the range so that the signal can penetrate through kilometres of ice sheet. The EastGRIP team hope to finish drilling their ice core in 2021, which will give unprecedented access to the bed of the North East Greenland Ice Stream (NEGIS) – a fast-moving area of glacier. We don’t fully understand why NEGIS moves so quickly, though it’s thought to be caused by geothermal heat warming the bed of the glacier and making it slide more quickly. We hope to deploy Cryoeggs into the NEGIS using EastGRIP’s borehole, which will give us the first direct observations of this fascinating environment, and help us understand how the conditions at its bed contribute to its rapid motion. Watch this space!
Further reading
- If you would like to know more about Cryoegg and our field trials – please read our preprint: https://doi.org/10.31223/osf.io/btphy
- Röthlisberger H and Lang H (1987) Glacial Hydrology. Glacio-Fluvial Sediment Transfer: An Alpine Perspective. Wiley, 207–84
Edited by Jenny Arthur
Mike Prior-Jones is a post-doctoral researcher at the School of Earth and Ocean Sciences at Cardiff University, working with glaciologist Liz Bagshaw. He is an electronic engineer with a particular interest in wireless communications. He has worked both in commercial engineering and in polar research, including field trips to both the Arctic and Antarctic. He is a member of Pride in Polar Research (@PridePolar), a network for LGBTQIA+ people in polar research. He tweets as @DrMikePJ.
Contact Email: Prior-JonesM@cardiff.ac.uk