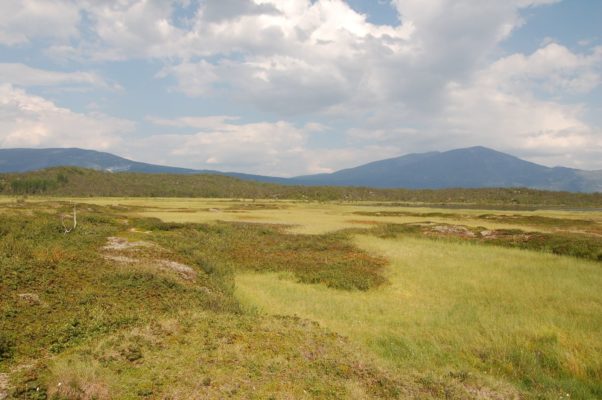
Permafrost, or perennially frozen, peatlands are among the world’s largest terrestrial carbon stores and are particularly threatened by warming climates. Understanding how modern climate controls the distribution of permafrost peatlands is crucial for making confident predictions of their past and future extents.
What are permafrost peatlands?
Peatlands are wetlands that develop where cold, wet conditions prevent deceased organic matter from fully decomposing, producing organic-rich soils known as peat. Peatlands are widely distributed across the northern hemisphere, with recent estimates suggesting that northern peatlands cover ~2.8 million km2.
The majority of northern peatlands are located within the permafrost zones of Canada, Alaska, northern Europe, and Siberia. These peatlands contain varying amounts of permafrost – ground that has been frozen for two or more consecutive years. Whilst frozen, permafrost peatlands often exist as inert carbon stores because surrounding cold, dry climates restrict both carbon sequestration (the capture and storage of atmospheric carbon, e.g. through reduced plant productivity) and peat decomposition (e.g. inhibiting microbial respiration). Peatlands within the northern permafrost zone store vast amounts of soil organic carbon, estimated at ~277 Pg, which equates to a third of the total carbon presently found in our atmosphere.
In recent decades, permafrost thaw has been widely observed across the northern hemisphere. Peatland surfaces commonly become saturated following permafrost thaw, which creates conditions that encourage soil organic carbon to be preferentially released as methane, a greenhouse gas with ~28–34 times the global warming potential of carbon dioxide over 100 years. Widespread release of methane from thawing peatlands has the potential to rapidly accelerate climate change. However, predicting how the permafrost peatland carbon store will be affected overall by future climate change remains highly complex. For example, rising temperatures will also produce longer, warmer growing seasons that may increase carbon intake by increasing plant productivity. At present, too many uncertainties persist for scientists to make confident predictions as to how this complex balance will play out over the coming decades.
The distribution of permafrost peatlands remains poorly constrained
A key knowledge gap that complicates such predictions is that the distribution of permafrost peatlands remains poorly constrained for much of the northern hemisphere. It is therefore unclear when and where changes may be expected to occur. A substantial amount of research has taken place in Fennoscandia, northern Europe, to map the distribution of permafrost peatlands through aerial imagery and field observations. This has facilitated the development of statistical models that quantify the climatic conditions that maintain permafrost peatlands in this region.
The distribution of permafrost peatlands was particularly poorly known in northern North America up until recently. This was partly because North American permafrost peatlands are predominately found in highly remote and inaccessible regions of northern Canada and Alaska where fieldwork is made extremely challenging. Over recent decades, however, scientific interest in these regions has substantially increased, and there are now sufficient records of North American permafrost peatlands within the scientific literature for meaningful statistical analyses.
Palsas
One of the challenges in mapping permafrost distribution is that frozen ground is inherently a subsurface entity (i.e. hidden underground). The presence of permafrost can only be identified within a peatland either by sampling sediments beneath the surface, or by identifying tell-tale surface landforms. One such landform are palsas (Figure 1), frozen mounds within peatlands. Palsas form when permafrost ice lenses expand beneath the peat surface, causing the surface to rise. A recent blog post provides a more detailed description of these landforms. Palsas are particularly useful for building distribution maps of permafrost peatlands because they are easily identifiable in aerial imagery, are highly climatically sensitive, and can exist right up to the southernmost extent of the permafrost zone.
Our research
In our recent study we aimed to statistically model, for the first time, the range or ‘envelope’ of climatic conditions that currently maintain palsas across North America. We began by constructing the largest catalogue of North American palsas currently available, with over 350 unique sites (Figure 2). We then fitted a binary (i.e. palsas present or palsas absent) statistical model to relate landform presence to modern climate data.
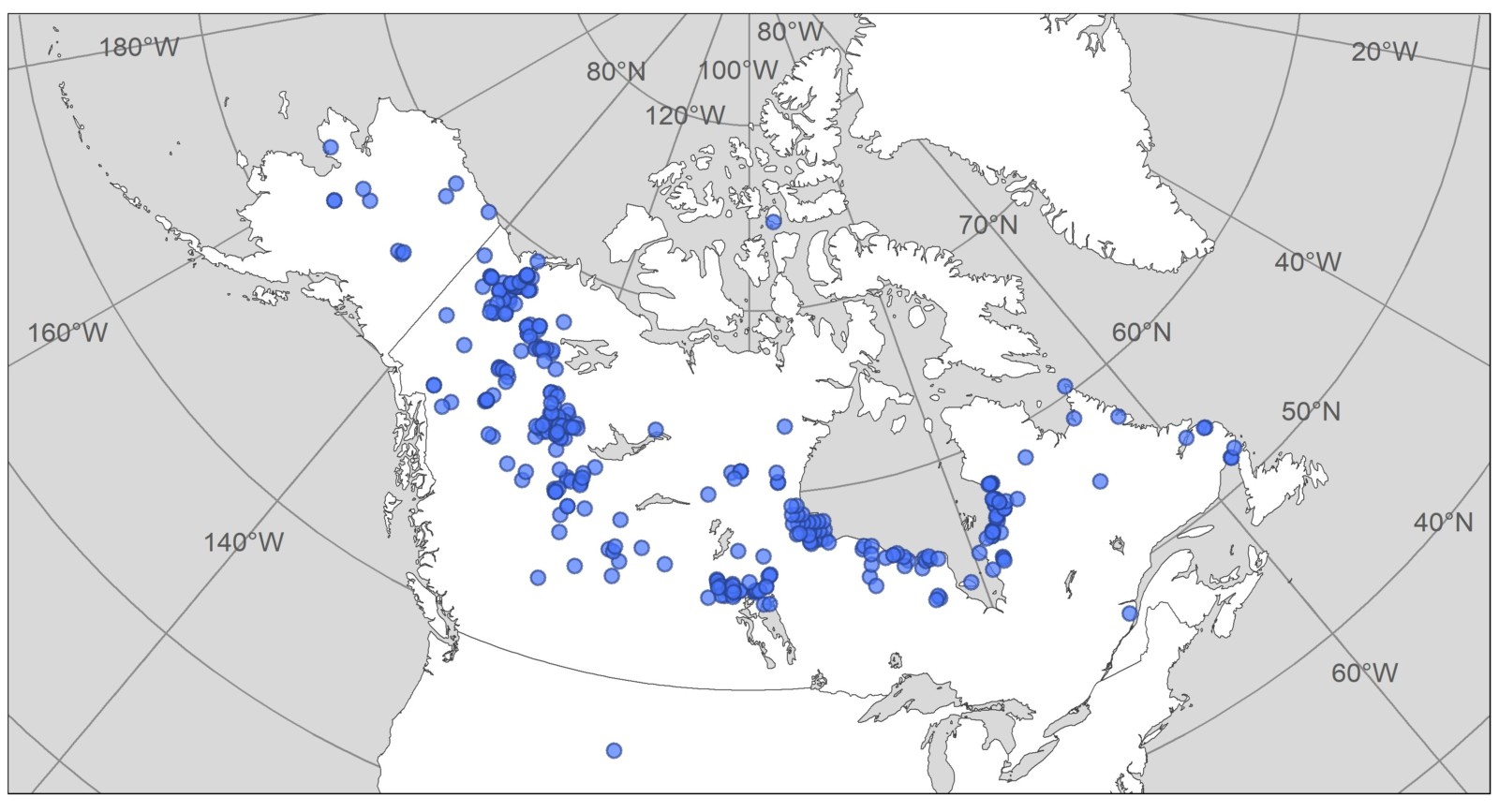
Figure 2. The locations of the 352 North American palsas (blue dots) used to fit the climate envelope model in Fewster et al. (2020).
The distribution of modern North American palsas was found to be significantly related to several climate variables: mean annual temperature; annual rainfall; growing degree days (the annual time integral of temperatures above 5°C); and the annual temperature range. Our model indicates that North American palsas favour cold, dry climates with mean annual temperatures between -11.3°C and 0.19°C and with annual rainfall of between 112 and 506 mm per year. This climate envelope is broader than those previously reported for palsas in northern Europe. A few observed palsa sites were not predicted by our model. These sites correspond to more extreme climates where local factors, such as vegetation cover and topography, alter the local conditions. But our model performed well overall and correctly predicted ~85 % of the observed landforms (Figure 3).
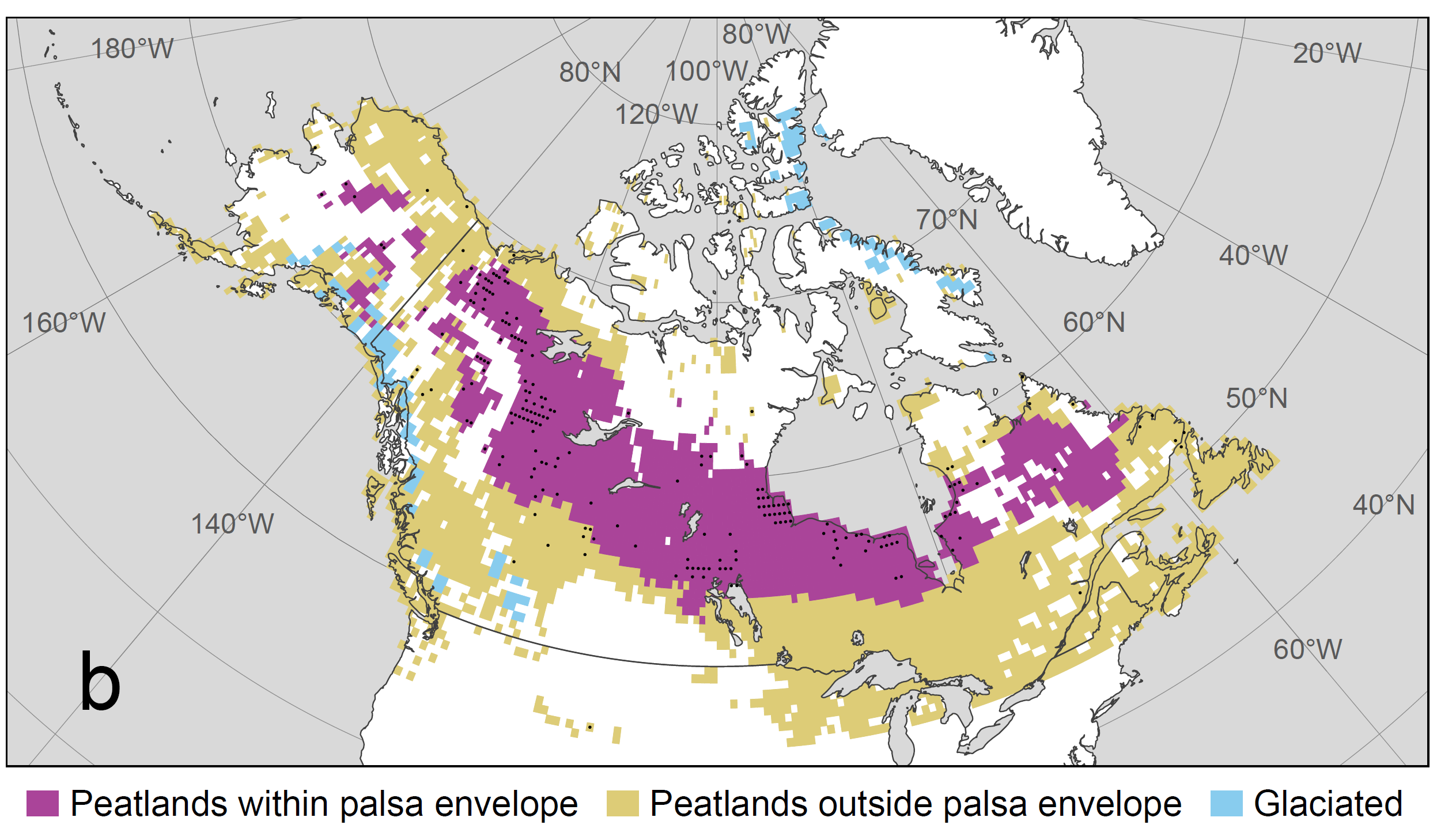
Figure 3. Predicted distribution of the suitable climate envelope for palsas in North America, presented as a map of peatlands classified as those that are climatically suitable for palsas (in purple), and peatlands that are climatically unsuitable for palsas (in yellow). Black dots represent grid cells that contain observations of palsas. See Fewster et al. (2020) for further details.
Simulations of past distributions
As a second stage of our research, we used our climate envelope model to simulate possible past distributions of North American palsas throughout the Holocene (a time period spanning the last 11.5 thousand years) at 500 year intervals using simulations of past climate generated from a general circulation model. We found that it was also important to account for several non-climatic factors when simulating past palsa distributions. For example, the North American landscape was once dominated by the presence of vast ice sheets (e.g. the Laurentide Ice Sheet) and post-glacial water bodies (e.g. Lake Agassiz), which restricted the land area available for palsas to form. Peatlands were also less widely distributed 11.5 thousand years ago than today. We therefore used dates of deglaciation, terrestrial submergence (e.g. flooding following the expansion of post-glacial lakes) and peatland initiation to constrain our Holocene simulations to only grid cells that were environmentally plausible at each modelled time step.
Our final results suggest that suitable climatic conditions for palsa presence developed much earlier in Alaska and northwestern Canada than in eastern Canada, which was a region dominated by the Laurentide Ice Sheet for much of the Holocene (Figure 4). Late deglaciation delayed peatland initiation in eastern Canada and prevented suitable climatic conditions for palsa development until ~6000 years ago. We found that our results broadly agree with existing evidence from peat core studies.
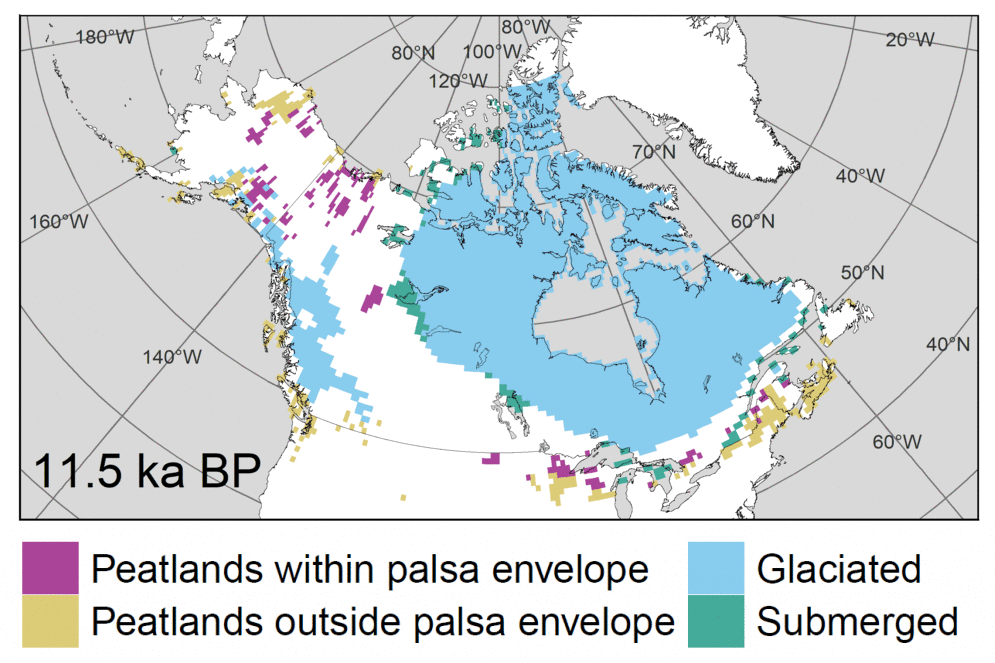
Figure 4. Predicted Holocene distribution of North American palsas (shown in purple), once filtered for the presence of peat, and the absence of continental ice and postglacial water bodies. See Fewster et al. (2020) for full details.
What does this mean for the future?
Palsa thaw in response to recent climate change has been widely observed across North America (e.g. Payette et al., 2004; Mamet et al., 2017), particularly at their most southerly limit. As temperature and precipitation are projected to increase across the northern hemisphere over the coming decades, we may expect the southern extent of palsas to shrink further northwards by 2100. Some more northerly locations, such as arctic deserts, may ultimately become more climatically suitable to palsas, but our Holocene simulations indicate that the timescales required for the formation of suitable environmental conditions will take substantially longer (e.g. the time required for peat to become deep enough to insulate ice lenses). We can tentatively expect, therefore, that the spatial extent of palsas in North America will reduce by 2100.
Further research is required, however, to more confidently understand when, where, and how these changes will take place, which is critical for predicting the true impact of future climate change on this vast, but vulnerable, organic carbon store. Our new catalogue of North American palsa distributions and climate envelope modelling will make an important contribution to this ongoing endeavour.
Further reading
To learn more about this research, please see the full article ‘Drivers of Holocene palsa distribution in North America’ (Fewster et al., 2020) here:
- Fewster, R.E., Morris, P.J., Swindles, G.T., Gregoire, L.J., Ivanovic, R.F., Valdes, P.J. and Mullan, D. 2020. Drivers of Holocene palsa distribution in North America. Quaternary Science Reviews. 240, 106337. www.sciencedirect.com/science/article/pii/S0277379120302997
- Luoto, M., Fronzek, S. and Zuidhoff, F.S. 2004. Spatial modelling of palsa mires in relation to climate in northern Europe. Earth Surface Processes and Landforms. The Journal of the British Geomorphological Research Group. 29(11), pp.1373-1387. https://doi.org/10.1002/esp.1099
- Mamet, S.D., Chun, K.P., Kershaw, G.G., Loranty, M.M. and Kershaw, G.P. 2017. Recent increases in permafrost thaw rates and areal loss of palsas in the Western Northwest Territories, Canada. Permafrost and periglacial processes. 28(4), pp.619-633. https://doi.org/10.1002/ppp.1951
- Payette, S., Delwaide, A., Caccianiga, M. and Beauchemin, M. 2004. Accelerated thawing of subarctic peatland permafrost over the last 50 years. Geophysical research letters. 31(18). https://doi.org/10.1029/2004GL020358
- Tarnocai, C., Canadell, J.G., Schuur, E.A., Kuhry, P., Mazhitova, G. and Zimov, S. 2009. Soil organic carbon pools in the northern circumpolar permafrost region. Global biogeochemical cycles. 23(2). https://doi.org/10.1029/2008GB003327
- Treat, C.C. and Jones, M.C. 2018. Near-surface permafrost aggradation in Northern Hemisphere peatlands shows regional and global trends during the past 6000 years. The Holocene. 28(6), pp.998-1010. https://doi.org/10.1177%2F0959683617752858
Edited by Keir Nichols and Marie Cavitte
Richard Fewster is a PhD student in the School of Geography at the University of Leeds, UK. He researches the past, present and future development of permafrost peatlands using statistical modelling, palaeoecology and fieldwork-based approaches. He tweets as @richard_fewster. Contact email: gy15ref@leeds.ac.uk.