It’s pitch black and twenty degrees below zero; so cold that the hairs in your nose freeze. The Arctic Ocean in autumn and winter is inhospitable for both humans and most scientific equipment. This means there are very few close-up observations of sea ice made during these times.
Recently, rapidly declining coverage of sea ice in the Arctic Ocean due to warming climate and the impending likelihood of an ‘ice-free Arctic’ have increased research and interest in the polar regions. But despite the warming trends, every autumn and winter the polar oceans still get cold, dark, and icy. If we want to truly understand how sea ice cover is evolving now and into the future, we need to better understand how it is growing as well as how it is melting.
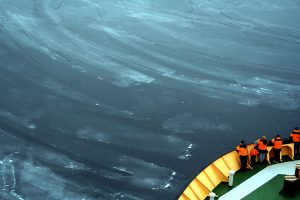
Nilas or thin sheets of sea ice [Credit: Brocken Inaglory (distributed via Wikimedia Commons) ]
Sea ice formation
Sea ice formation during the autumn and winter is complex. Interactions between ocean waves and sea ice cover determine how far waves penetrate into the ice, and how the sea ice forms in the first place. If the ocean is still, sea ice forms as large, thin sheets called ‘nilas’. If there are waves on the ocean surface, sea ice forms as ‘pancake’ floes – small circular pieces of ice. As the Arctic transitions to a seasonally ice-free state, there are larger and larger areas of open water (fetch) over which ocean surface waves can travel and gain intensity. Over time, with the continued action of waves in the ice, pancake ice floes develop raised edges — as seen in our image of the week — from repeatedly bumping into each other. Pancake ice is becoming more common in the Arctic, and it is already very common in the Antarctic, where almost all of the sea ice grows and melts every year.
Nilas vs pancakes
Nilas and pancake sea ice are different at the crystal level (see previous post), and regions of pancake ice and nilas of the same age may have different average ice thickness and ice concentration. As a result, the interaction of the ocean and atmosphere in these two ice types may be very different. Gaps of open water between pancake ice floes allow heat fluxes to be exchanged between the ocean and atmosphere – which can have very different temperatures during winter. Nilas and pancakes also interact with waves differently – nilas might simply flex with a low-intensity wave field, or break into pieces if disturbed by large waves, while pancakes bob around in waves, causing a viscous damping of the wave field. The two ice types have very different floe sizes (see previous posts here and here). Nilas is by definition is a large, uniform sheet of ice; pancake floes are initially very small and grow laterally as more frazil crystals in the ocean adhere to their sides, and multiple floes weld together into sheets of cemented pancakes.
How to make observations?
Sea ice models have only recently begun to be able to separate different sizes of sea ice. This allows more accurate inclusion of growth and melt processes that occur with the different sea ice types. However, observations of how sea ice floe size changes during freeze-up are required to inform these new models, and these observations have never been made before. Pancake sea ice floes are often around only 10 cm in diameter initially, which is far too small to observe by satellite. This means that observations of pancake growth need to be made close-up, but the dynamic ocean conditions in which pancakes are created makes it difficult to deploy instruments in-situ. So how can we observe pancake sea ice in this challenging environment?
In a recent paper (Roach et al, 2018), we used drifting wave buoys, called SWIFTs, to capture the growth of sea ice floes in the Arctic Ocean. SWIFTs are unique platforms (see image of the week) which drift in step with sea ice floes, recording air temperature, water temperature, ocean wave data and – crucially for sea ice – images of the surrounding ice. Analysis of the series of images captured has provided the first-ever measurements of pancake freezing processes in the field, giving unique insight into how pancake floes evolve over time as a result of wave and freezing conditions. This dataset has been compared with theoretical predictions to help inform the next generation of sea ice models. The new models will allow researchers to investigate whether describing physical processes that occur on the scale of centimetres is important for prediction of the polar climate system.
Edited by Sophie Berger
Lettie Roach is a PhD student at Victoria University of Wellington and the National Institute for Water and Atmospheric Research in New Zealand. Her project is on the representation of sea ice in large-scale models, including model development, model-observation comparisons and observation of small-scale sea ice processes.
Maddie Smith is a PhD student at the Applied Physics Lab at the University of Washington in Seattle, United States. She uses observations to improve understanding of air-sea interactions in polar, ice-covered oceans.